[ad_1]
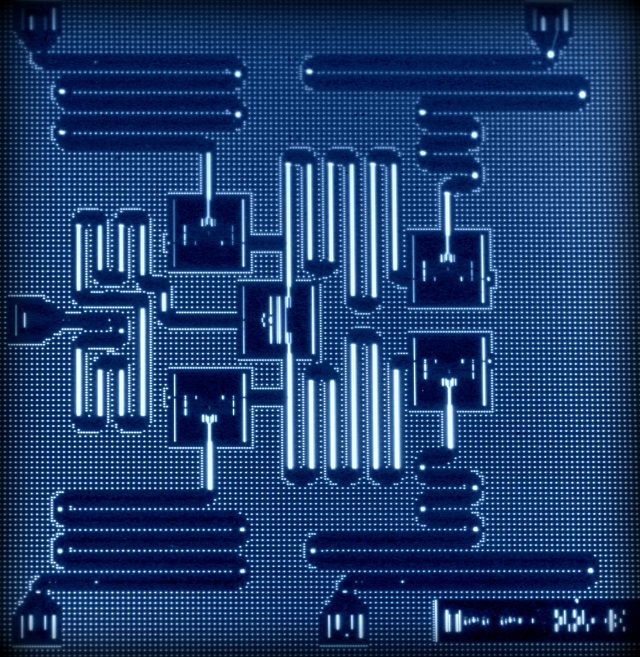
An image of the IBM quantum computer showing five qubits.
IBM
If I currently compared quantum computing to classical computing, I would say we are somewhere beyond the point where Babbage and Lovelace have stopped but have not yet reached the point where One made a Bomba or an ENIAC. We are currently trying to determine which technology is best for implementing a quantum computer. And this choice is not simple.
A technological choice for quantum computing is the ion trapped. The challenge is to get the largest possible number of ions under our control in a trap. As a rule, it means about ten ions, but we need more for useful calculations. This is a big step forward in the report on cooling a 2D crystal of nearly 200 ions to the lowest possible temperature.
Floating checkerboard of ions
An ion, for our purposes, is an atom with an electron removed. Because of the missing electron, each ion has a positive charge, so the ions repel each other. Likewise, the positive charge means that they can be fenced with electric and magnetic fields. When the ion traps are properly constructed, the ions self-organize into crystals – ions spaced apart from each other at regular intervals. In quantum computing, these crystals are limited to 1D, a chain of ions spaced equally.
A long chain of 1D ions is rather difficult to handle. The length of the trap becomes its Achilles heel: the ions at the ends of the trap behave differently than those in the center. This difference makes life difficult for anyone trying to use them to calculate.
Enter the 2D crystal. A 2D crystal has all the advantages of a 1D crystal but is a bit more compact. We have not used 2D crystals yet, because we can not cool them easily. If the crystal is not cold, the quantum information stored in the ions will be quickly destroyed.
Blinded in neutral
To understand how physicists cool ions and atoms to near absolute zero, it is useful to visualize ions in the same way as physicists do. As shown below, the ion is nothing but its possible energetic states. In this diagram, we show four possible states. All ions start in one energy state. However, as all ions are in motion, the state is unclear: all ions have a slightly different energy than an immobile ion.
Chris Lee
Most cooling systems use the Doppler effect. The Doppler effect is what makes the siren of an ambulance approach more serious than when the ambulance is moving away.
For ions, it is useful to think of Doppler cooling compared to its opposite. An ion that is do not moving can absorb a certain color of light. By absorbing a photon, the ion kicks and begins to move away from the light source. Since it's moving, light from the same fixed source is no longer the right wavelength to absorb. The next photon should be slightly blueer to be absorbed by the ion due to the Doppler shift. If you make the light source slightly bluer, the ion gets another kick and moves slightly faster: we heat the ion.
We can reverse this process, as shown below, using a slightly redder light. The only ions that absorb light move toward the light source – the Doppler shift makes the light blue for these ions, allowing it to be absorbed. The absorption of the photon slows down the ion.
Chris Lee
But the Doppler shift can only take you far. At one point, the effect of heating the light shifted to blue equilibrium with the cooling effect of the light shifted to red to prevent further cooling. At this point, we need a mechanism to selectively prevent warming of cold ions.
That the game is hiding the ion
Hiding the ion is basically what researchers do. The ions they use have many different quantum states. The laser that performs the Doppler cooling moves them between two states. One of these two states is then coupled via a pair of lasers to a third state.
As shown in the illustration below, the additional pair of lasers is also not well suited to the energy levels of the ions. The combination of their brightness and this slight difference in energy deforms the energy levels of the ions so that the Doppler cooling laser can only slow the ions moving faster and not accelerate the slow moving ions. In other words, this allows the selection of warmer ions.
Chris Lee
As a result, the ionic crystal cools to a lower temperature. How cold? The researchers measured the energy contained in the collective movement of ions (called "drumhead" mode) and showed that about 70% of the time, the ions were in the lowest possible state (for a movement in one direction data). This is tantamount to a kind of failure because, as the researchers point out, they should be able to get the lowest possible state 95% of the time. They do not know why their results do not fit the theory.
Yet, this is exactly the progress required to obtain 2D ion crystals ready for the intoxicating world of quantum computing.
Letters of physical examination, 2019, DOI: 10.1103 / PhysRevLett.122.053603 (About DOIs)
[ad_2]
Source link