[ad_1]
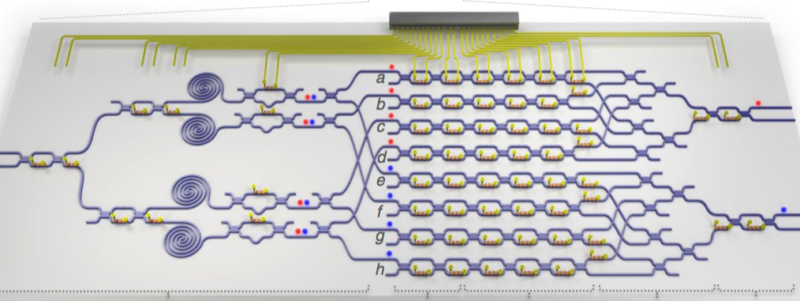
There is a moment in the life of every physicist when they think the unthinkable: I would like to be an engineer. I think this thought went through the minds of 14 physicists involved in creating a key demonstration of quantum computing's scalability using light.
At the present time, if you were to bet on the technology most likely to win the race for quantum computing, most people would put their chips on a series of superconducting rings. But I put the house and the children in the light. Why? Because lasers improve everything. More seriously, quantum computer architectures based on superconducting devices have made remarkable progress over the past five to ten years. On the other hand, progress on the light front has been terribly slow. But it should be easier to work with light qubits if we can get them off the ground.
Why do I like photons
As far as I'm concerned, photons always produce the best quantum bits (qubits). This is because photons cross the world unhindered. A photon, in a super – special quantum state, can pass from the air to an optical fiber to pass through the air, through a silicon chip, in the air and in a fiber, without destroying its quantum state. About everything you need to make sure your photon detector is in the dark so that only the qubit photons touch it.
Superconducting qubits consist of electrons, sensitive to everything. It takes real experimental skill and good engineering to ensure that superconducting qubits retain their quantum state.
You should be wondering why, if the light is so good, quantum computers based on light are so far behind. It's exactly for the same reason: photons do not notice, but electrons do. Imagine that you want to change the state of one qubit according to the state of another qubit. For electrons, it's simple because they have an electric and magnetic field through which they can be manipulated. Photons, however, just pass each other without realizing. The simple way to implement quantum operations is actually very, very difficult using photons.
Instead, you must use multiple combinations of linear operators. What is the difference? In other words, in a non-linear operation, two photons can collide to create a single photon, or a single photon can split to create two photons. But, in a linear operation, the number of photons is preserved and only their paths are modified.
To perform a two-qubit operation, you need at least one door and three qubits, two that are used and one that controls the operation. To perform the same operation using only linear operations (required for photons) requires four qubits and four gates. The complexity is increasing rapidly.
This is where the shift from physics to engineering is needed. Implementing a single door is complex but feasible. But, what about the implementation of all possible doors for, say, two qubits? This requires the design of a custom integrated optical chip. It is here that the engineers intervene.
The chip produced by the researchers is quite remarkable. It takes a single source of laser light and, from there, generates pairs of photonic qubits. The qubits then pass through a single gate made up of a labyrinth of interferometers (linear operation used to construct the gates). Each waveguide has a small heating element that allows researchers to control the exact distance between photons and each interferometer. This control determines the path traveled by the photons in the labyrinth. Or more precisely, the control, combined with the quantum state of the photon, determines the trajectory through the labyrinth.
Researchers have demonstrated this by implementing 98 gates at two different qubits on the same hardware. And, with each, they performed a complete set of measurements (about 1,000 measurements per door). The doors are about as reliable as any other you will find in the world of quantum computing, that is, the operations succeed in 93% of the cases. In comparison, quantum computers with ions are 95 to 99% and quantum computers are about 90 to 95% superconducting.
To show that the chip was capable of more than just a simple operation, the researchers showed that you could run an optimization algorithm.
Two-bit paradise
It's still only two qubits, which is nothing compared to superconducting quantum computers, which are now in the 20-bit range. So, why am I excited? The fact is that this article shows that a lot of big problems have been overcome. Researchers have shown that you can design, manufacture and control a chip with the precision required to programmable quantum computing. There is not much to prevent the door design from moving to more elaborate circuits that could run larger programs (if the chip had enough qubits and they could be detected) .
There are some potential stumbling blocks. The photon detectors were not on the chip. Instead, the light was routed to external detectors. For two qubits (just two external detectors), this is achievable. For 100 qubits, it probably will not work. It may be necessary to stick two chips, one for the calculation and the other for the detection.
Likewise, the light source will be difficult. The researchers' production process for entangled photons is a random process. Each laser pulse may or may not generate entangled photons. In the case of this chip, which has only two calculation qubits, there are four independent locations where these photons are generated. For a calculation to take place, two locations must produce a pair of photons independently. This occurs for about a quarter of the laser pulses.
To increase the number of entangled photons, you must generate them simultaneously on several sites. The chances of this happening are not great; a computer of 100 qubits would never be expected to work. There are, I should say, many other ways to produce the required photons. And many of them are deterministic: you press a button and get a photon. But the big question is whether they can be integrated with the chip technology developed here.
In any case, I am very excited about developments on the calculations side. And very suspicious of the road ahead to generate the required photons and detect the result of the calculation.
Photonic Nature, 2018, DOI: 10.1038 / s41566-018-0236-y. (About DOIs)
Source link