[ad_1]
The energy-providing bacterial endosymbiont allows its unicellular eukaryotic host to breathe nitrate, demonstrating that unicellular eukaryotes can acquire endosymbionts to supplement or replace the functions of their mitochondrial organelles.
Researchers in Bremen, along with their colleagues at the Max Planck Genome Center in Cologne and the Eawag Aquatic Research Institute in Switzerland, have discovered a unique bacterium that lives inside a single-celled eukaryote and provides it with energy . Unlike mitochondria, this so-called endosymbiont derives its energy from nitrate respiration, not oxygen. “Such a partnership is completely new,” says Jana Milucka, lead author of the Nature paper. “A symbiosis based on respiration and energy transfer is unprecedented to this day.”
In general, among eukaryotes, symbioses are quite common. Eukaryotic hosts often coexist with other organisms, such as bacteria. Some bacteria live inside host cells or tissues and provide certain services, such as defense or nutrition. In return, the host provides suitable shelter and living conditions for the symbiote. An endosymbiosis can even go so far that the bacteria loses its ability to survive on its own outside its host.
This was also the case with the symbiosis discovered by scientists from Bremen in Lake Zug in Switzerland. “Our discovery opens up the possibility that simple unicellular eukaryotes, such as protists, can harbor energy-providing endosymbionts to supplement or even replace the functions of their mitochondria,” explains Jon Graf, first author of the study. “This protist has managed to survive without oxygen by associating with an endosymbiont capable of breathing nitrates.” The name of the endosymbiont “Candidatus Azoamicus ciliaticola” reflects this; a “nitrogen friend” who lives in a ciliate.
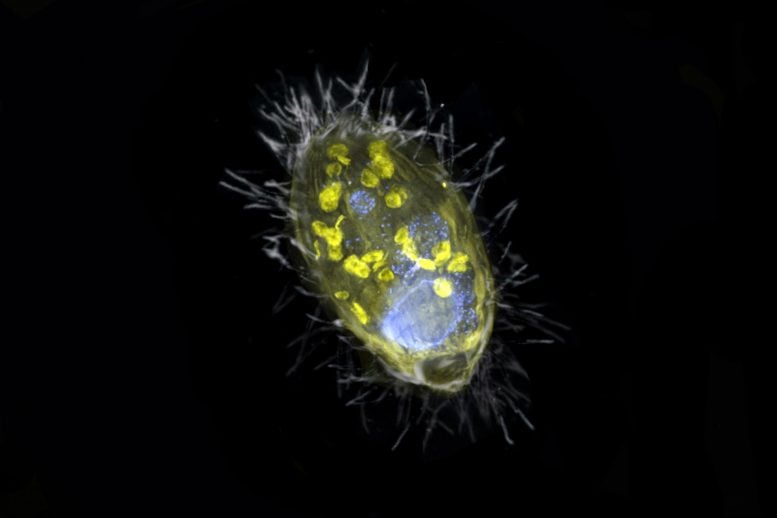
The figure is a composite of a scanning electron microscope image (SEM, gray) and fluorescence images. The endosymbiont ‘Candidatus Azoamicus ciliaticola’ (visualized by FISH, yellow) and bacterial prey in food vacuoles as well as the nucleus of large cells (stained by DAPI, blue) are visible. The external structure of the weakly fluorescent ciliate as well as the eyelashes are also visible. Credit: Max Planck Institute for Marine Microbiology, S. Ahmerkamp
An intimate partnership is getting closer and closer
Until now, it has been assumed that eukaryotes in oxygen-free environments survive through fermentation because mitochondria need oxygen to generate energy. The fermentation process is well documented and has been observed in many anaerobic ciliates. However, microorganisms cannot get as much energy from fermentation, and they usually don’t grow and divide as quickly as their aerobic counterparts.
“Our ciliate found a solution for this,” says Graf. “He swallowed up a bacterium capable of breathing nitrate and integrated it into his cell. We estimate that assimilation took place at least 200 to 300 million years ago. Since then, evolution has further deepened this intimate partnership.
Evolution over time
The evolution of mitochondria took place in a similar fashion. “All mitochondria have a common origin,” explains Jana Milucka. It is believed that over a billion years ago, when an ancestral archeon engulfed a bacterium, these two began a very important symbiosis: this event marked the origin of the eukaryotic cell. Over time, the bacteria became more and more integrated into the cell, gradually reducing its genome. Properties that were no longer needed were lost and only those that benefited the host were retained. Eventually, mitochondria evolved as we know them today. They have their own tiny genome as well as a cell membrane and exist as so-called organelles in eukaryotes. In the human body, for example, they are present in almost all cells and provide them – and therefore us – with energy.
“Our endosymbiont is capable of performing many mitochondrial functions, even if it does not share a common evolutionary origin with the mitochondria,” explains Milucka. “It’s tempting to speculate that the symbiote could follow the same path as the mitochondria, and eventually become an organelle.
A chance encounter
It is actually astonishing that this symbiosis has remained unknown for so long. Mitochondria work so well with oxygen – why wouldn’t there be an equivalent for nitrate? One possible answer is that no one was aware of this possibility and therefore no one was looking for it. The study of endosymbiosis is difficult because most symbiotic microorganisms cannot be cultured in the laboratory. However, recent advances in metagenomic analyzes have given us a better understanding of the complex interaction between hosts and symbionts. When analyzing a metagenome, scientists look at all the genes in a sample. This approach is often used for environmental samples because the genes in a sample cannot be automatically assigned to the organisms present. This means that scientists typically look for specific gene sequences that are relevant to their research question. Metagenomes often contain millions of different gene sequences and it is quite normal that only a small fraction of them are analyzed in detail.
Originally, scientists in Bremen were also looking for something else. The greenhouse gas research group at the Max-Planck-Institute for Marine Microbiology studies the microorganisms involved in the metabolism of methane. For this, they studied the deep layers of Lake Zug. The lake is very stratified, which means that there is no vertical water exchange. The deep layers of Lake Zug therefore have no contact with surface water and are largely isolated. This is why they do not contain oxygen but are rich in methane and nitrogenous compounds, such as nitrate. While searching for methane-munching bacteria with genes for nitrogen conversion, Graf came across a surprisingly small gene sequence that encoded the entire metabolic pathway for nitrate respiration. “We were all stunned by this discovery and I started to compare the DNA with similar gene sequences in a database, ”says Graf. But the only similar DNA belonged to that of the symbionts that live in aphids and other insects. “It didn’t make sense. How would insects get into these deep waters? And why? Graf remembers. Scientists in the research group began to guess games and bets.
Not alone in the dark lake
In the end, one thought prevailed: the genome must belong to an as yet unknown endosymbiont. To verify this theory, members of the research team undertook several expeditions to Lake Zug in Switzerland. With the help of local cooperation partner Eawag, they collected samples to specifically research the organism that contains this unique endosymbiont. In the lab, scientists extracted various eukaryotes from the water samples with a pipette. Finally, using a genetic marker, it was possible to visualize the endosymbiont and identify its protist host.
A final excursion a year ago was supposed to bring the final certainty. It was a difficult undertaking in the dead of winter. Stormy weather, dense fog and time pressure from the first coronavirus news along with a possible lockdown made searching the large lake even more difficult. Nevertheless, scientists managed to collect several samples from the deep waters and bring them to Bremen. These samples gave them the definitive confirmation of their theory. “It’s good to know that they are there together,” says Jana Milucka. “Normally, these ciliates eat bacteria. But he left one alive and joined forces with him.
Lots of new questions
This discovery raises many new and exciting questions. Are there similar symbioses that have been around much longer and where the endosymbiont has already crossed the border into an organelle? If such a symbiosis exists for the respiration of nitrates, does it also exist for other compounds? How did this symbiosis, which has existed for 200 to 300 million years, end up in a post-glacial lake in the Alps that only formed 10,000 years ago? In addition: “Now that we know what we are looking for, we have found the genetic sequences for endosymbiont all over the world,” says Milucka. In France, as well as in Taiwan or in the lakes of East Africa which are partly much older than Lake Zug. Does the origin of this symbiosis lie in one of them? Or did it start in the ocean? These are the questions the research group wishes to study next.
Reference: March 3, 2021, Nature.
DOI: 10.1038 / s41586-021-03297-6
[ad_2]
Source link