[ad_1]
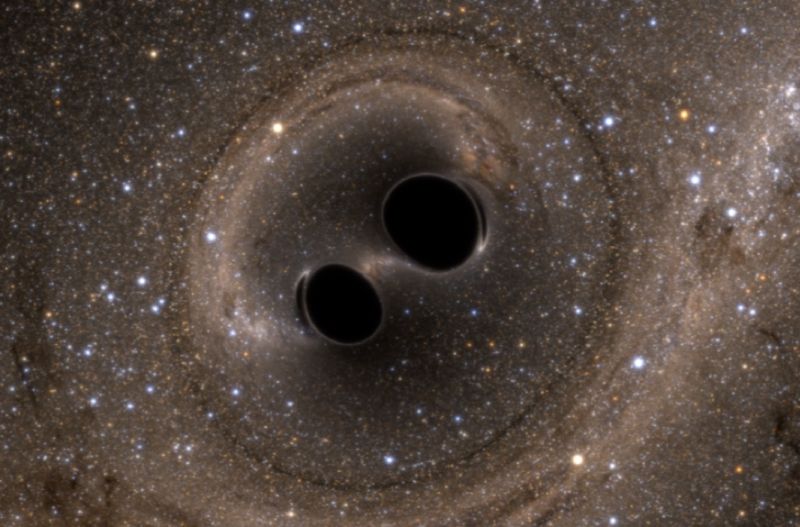
Physicists have "heard" for the first time the ringing of a hole in the back of an infant, thanks to a new analysis of LIGO data. Researchers have specifically sought revealing "connotations" in data from the Nobel Prize-winning collaboration in the fusion of two black holes. Not only were harmonics present, but the model of height and decay correspond to the predictions of black hole mass and spin derived from the general theory of relativity. According to a new article in Physical Review Letters, the result also corroborates the so-called "no hair" theorem for the classic description of black holes.
This classic image of a black hole is a circle with a point in the center. The circumference of the circle is the horizon of events and the point is the singularity. General relativity maintains that the area of the event horizon is a void without structure. Indeed, any dust, gas or elemental particle placed on the horizon should fall into the black hole, thus maintaining the vacuum. There would be no noticeable change if you cast something in a black hole – nothing that could provide a clue to what this object might have been. It's the late physicist John Wheeler who invented this colorful description: "Black holes do not have hair." (Wheeler had the knack for catchy names and phrases.) So it's enough to describe the black holes mathematically, it's their mass and rotation, plus their electrical charge.
"We all expect general relativity to be correct, but it is the first time we confirm it in this way," said the paper's senior author, Maximiliano Isi, of MIT. "This is the first experimental measure that allows to directly test the theorem of the absence of hair. This does not mean that black holes can not have hair. This means that the image of hairless black holes still lasts a day. "
"The image of black hairless holes is still alive one day."
General relativity also predicts that the fusion of two black holes should release powerful gravitational waves – ripples in the space-time structure so weak that they are very difficult to detect. LIGO is able to do this by laser interferometry using powerful lasers to measure minute changes in the distance between two objects placed miles away. LIGO has detectors in Hanford, Washington, and Livingston, LA. A third detector in Italy, Advanced VIRGO, was put online in 2016. Each instrument is so sensitive that it also captures small ambient vibrations, such as a rumbling freight train or a natural thermal current. vibrations in the detectors themselves. LIGO's collaboration strives to protect its instruments and minimize noise in its data.
On September 14, 2015 at 5:51 am Eastern Time, the two detectors picked up signals within a few milliseconds for the very first time. The waveforms of these signals serve as audio footprints – in this case, the evidence of two black holes spiraling one towards the other and merging into a massive collision event , sending powerful shock waves in space-time. Resume the signals was a breathtaking achievement and no one was surprised when the first direct observation of gravitational waves won the 2017 Nobel Prize in Physics.
An upgraded LIGO still detects various other candidate events. Although some opponents expressed doubts about this first event, two independent studies confirmed the detection last year, so the controversy was largely dispelled.
Tones in the ringdown
LIGO detected a revealing "chirp" pattern in the data as the two black holes spiraled before fusing to form a new black hole. This new black hole should have vibrated under the effect of the force of the impact, and these vibrations, called "saccades", would also have produced gravitational waves. But physicists had assumed that the vibrations would be far too weak to be detected by the LIGO instruments, given all the noise of the initial collision.
Matt Giesler, co-author of the study and graduate student at Caltech, realized that this pessimism was caused by a focus on the main vibratory frequency. But this is not the only signal present. The gravitational wave signal must have several frequencies that fade at different speeds (decay), each tone corresponding to a vibratory frequency of the new black hole.
"There is not a single tone, there are actually a lot of tones that make up that ringing signal," Giesler said. "We realized that one of these additional tones should be visible in existing public data." If they are present, they should provide accurate estimates of the mass and rotation of the new black hole.
Having these additional tones also makes it possible to test the "hairless" theorem, which requires two independent measurements to confirm mass and spin. "People had this idea of doing the test, but they still thought they were using the fundamental tone," said co-author Saul Teukolsky, a physicist at Caltech and Cornell University.
Mr. Isi / Massachusetts Institute of Technology
Giesler tried to perform the test with simulated data. He discovered that he could detect the overtones in the last few milliseconds of the signal, just after the spike in revealing tweets, and reported his results to Teukolsky.
"I was very excited and said:" We have to try this on real data, "Teukolsky said. Indeed, they were able to identify two distinct tones in the data and measure the height and the decay of each. Their calculations correspond to the previous measurements of the mass and the spin of the black hole.
"To be quite honest, it's the first time this is done, and it's not a very rigorous test," added Teukolsky, noting error bars on frequencies measured and the depreciation times. "It was really more to show that it could be done, people thought we had to wait 15 to 20 years, maybe even for detectors in space, before the detector accuracy was sufficient to look for this. We've shown that we can start doing this now. "As detector accuracy improves over the next few years, these tests should improve."
Proof of principle
Now that proof of principle is established, physicists will try to look for harmonics in the data of other events detected by LIGO. But there is no guarantee of success, even with the significant improvements made to the detector since 2017.
"This first detection is still the most powerful signal we have seen so far," said Professor Teukolsky. "We must see another at least as good as this one." And for that, they will have to depend on the vagaries of nature.
"The bigger and stronger the event, the more likely it is that LIGO can get these harmonics," said Caltech physicist Alan Weinstein, a member of LIGO Lab, who is not co-author of this latest study. . "With the first gravitational wave detection by LIGO, we have confirmed the predictions made by general relativity.Now, by looking for even lower harmonics and signals called higher order modes, we are looking for tests. more in-depth theory and even potential evidence of breaking it down. "
For example, "black hole spectroscopy may one day exclude the possibility that what we call black holes are actually" imitators "of black holes – compact objects conjectured as boson stars or gravestars without a horizon. 39, event horizon, "wrote Marric Stephens at Physics APS. "Their properties will appear as deviations from ringdown signatures predicted by general relativity." In other words, such objects might not "ring" in the same way, which would give physicists a chance to spot them.
The work once again shows how much LIGO data can be useful for studying many types of difficult questions in physics. "It's great that all the data is made public," said Teukolsky, even though his group is part of the LIGO collaboration and would have had access at any time. "Really, no matter who could have taken the data from this first event and do this analysis."
DOI: Physical Review Letters, 2019. 10.1103 / PhysRevLett.123.111102 (About DOIs).
[ad_2]
Source link