[ad_1]
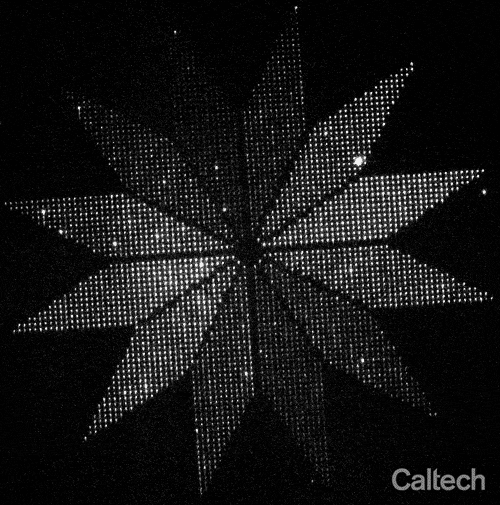
The researchers placed more than 3,000 shining moon-shaped nanoscale molecular devices in a flower-shaped instrument to indicate the polarization of light. The “moons” in each of the 12 petals point in a different direction and only glow when struck by polarized light corresponding to its orientation. The end result is a flower whose petals light up in sequence when the polarization of the light shining on it is rotated. The flower, which spans a distance less than the width of a human hair, demonstrates that thousands of molecules can be oriented reliably on the surface of a chip. Credit: Ashwin Gopinath / Caltech
The proof of concept project paves the way for the integration of molecules with computer chips.
Engineers have developed a technique that allows them to accurately place microscopic devices formed from DNA molecules in not only a specific location but also in a specific orientation.
As a proof of concept, they arranged more than 3,000 moon-shaped nanoscale molecular devices glowing in a flower-shaped instrument to indicate the polarization of light. Each of the 12 petals pointed in a different direction around the center of the flower, and inside each petal, approximately 250 moons were aligned in the direction of the petal. Because each moon only shines when struck by polarized light corresponding to its orientation, the end result is a flower whose petals light up in sequence when the polarization of the light shining on it is rotated. The flower, which spans a distance less than the width of a human hair, demonstrates that thousands of molecules can be oriented reliably on the surface of a chip.
This method of accurately placing and orienting DNA-based molecular devices may allow these molecular devices to be used to power new types of chips that integrate molecular biosensors with optics and electronics for applications such as DNA sequencing or measuring the concentrations of thousands of proteins at one time.
The research, published on February 19, 2021, by the journal Science, draws on over 15 years of work by Paul Rothemund (BS ’94) of Caltech, a research professor in bioengineering, computer science and mathematical sciences, computation and neural systems, and his colleagues. In 2006, Rothemund showed that DNA could be directed to fold into precise shapes using a technique called DNA origami. In 2009, Rothemund and his colleagues at IBM Research Almaden described a technique for positioning DNA origami at specific locations on surfaces. To do this, they used a printing process based on electron beams and created “sticky” patches that were the same size and shape as origami. In particular, they showed that the origami triangles bonded precisely to the location of the sticky triangle patches.
Next, Rothemund and Ashwin Gopinath, former Caltech senior postdoctoral researcher and now assistant professor at WITH, refined and extended this technique to demonstrate that molecular devices constructed from DNA origami can be reliably integrated into larger optical devices. “The technological barrier has been how to reproducibly organize a large number of molecular devices into the right models on the types of materials used for the chips,” says Rothemund.
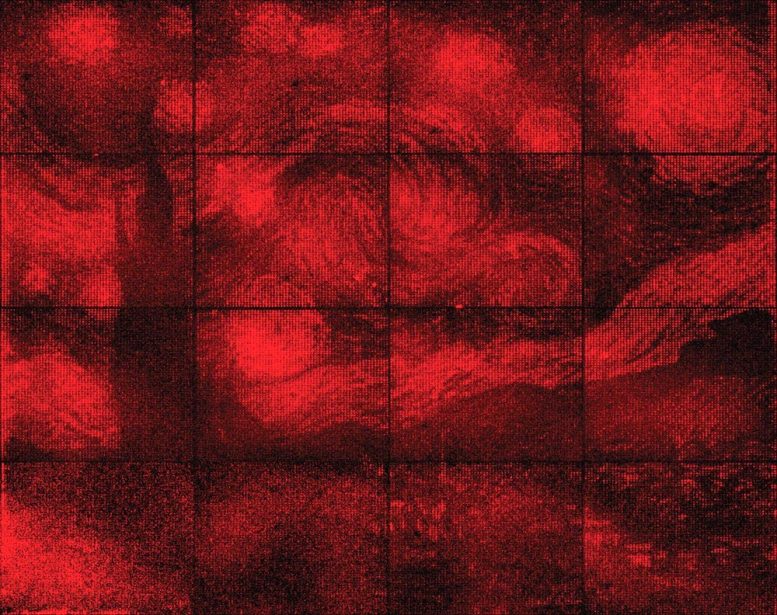
This vivid reproduction of “The Starry Night” contains 65,536 pixels and is the width of a penny. Credit: Ashwin Gopinath / Caltech
In 2016, Rothemund and Gopinath showed that triangular origami carrying fluorescent molecules could be used to replicate a 65,000 pixel version of Vincent van Gogh The starry Night. In this work, triangular DNA origami was used to position fluorescent molecules in bacteria-sized optical resonators; the precise placement of the fluorescent molecules was essential because a movement of just 100 nanometers to the left or right would dim or brighten the pixel more than five times.
But the technique had an Achilles heel: “Because the triangles were equilateral and were free to turn and turn inside out, they could stick flat to the triangular patch sticking to the surface in six different ways. This meant that we couldn’t use any device that required special orientation to work. We were stuck with devices that worked equally well when pointed up, down or in any direction, ”Gopinath explains. Molecular devices for DNA sequencing or protein measurement absolutely have to land upright, so the team’s older techniques would ruin 50% of the devices. For devices that also require a single rotational orientation, such as transistors, only 16% would work.
The first problem to be solved, then, was to land the DNA origami reliably with the correct side up. “It’s kind of like ensuring that toast always magically lands with the butter side up when it is thrown on the floor,” says Rothemund. To the researchers’ surprise, coating the origami with a carpet of flexible DNA strands on one side allowed more than 95% of them to land face up. But the problem of controlling the rotation remains. Right triangles with three different edge lengths were researchers’ first attempt at a shape that could land in the preferred rotation.
However, after struggling to get just 40% of the right triangles to point in the correct orientation, Gopinath recruited computer scientists Chris Thachuk from the Washington University, co-author of Science paper, and a former Caltech postdoc; and David Kirkpatrick of the University of British Columbia, also co-author of Science paper. Their job was to find a shape that would only get stuck in the right orientation, no matter what orientation it might land in. The computer scientists’ solution was a disc with an off-center hole, which the researchers called a “small moon.” “Mathematical evidence suggested that, unlike a right triangle, small moons could rotate smoothly to find the best alignment with their sticky patch without getting stuck. Laboratory experiments verified that over 98% of small moons found the correct orientation on their sticky plates.
The team then added special fluorescent molecules that clamp tightly into the DNA helices of small moons, perpendicular to the axis of the helices. This ensured that the fluorescent molecules inside a moon were all facing the same direction and would glow the brightest when stimulated by light of a particular polarization. “It’s like each molecule carries a small antenna, which can only accept the energy of light most efficiently when the polarization of the light matches the orientation of the antenna,” Gopinath explains. It is this simple effect that allowed the construction of the polarization sensitive flower.
With robust methods for controlling the upward and rotational orientation of DNA origami, a wide range of molecular devices can now be inexpensively integrated into high throughput computer chips for a variety of potential applications. For example, Rothemund and Gopinath founded a company, Palamedrix, to commercialize the technology for constructing semiconductor chips that allow the simultaneous study of all proteins relevant to human health. Caltech has filed patent applications for the work.
Reference: “Absolute and Arbitrary Orientation of Single-Molecule Forms” by Ashwin Gopinath, Chris Thachuk, Anya Mitskovets, Harry A. Atwater, David Kirkpatrick and Paul WK Rothemund, February 19, 2021, Science.
DOI: 10.1126 / science.abd6179
Caltech AUTHORS: 20181029-101527551
The article is titled “Absolute and Arbitrary Orientation of Single Molecular Forms”. Caltech co-authors include Harry Atwater, Howard Hughes Professor of Applied Physics and Materials Science, and former graduate student Anna Mitskovets (PhD ’20). This work was supported by the Office of Naval Research, the Air Force Office of Scientific Research, the National Science Foundation, the Orr Family Foundation, the Abedin Institute, and a Banting Postdoctoral Fellowship.
[ad_2]
Source link