[ad_1]
On February 22, a Falcon rocket took off from Cape Canaveral carrying the Indonesian communications satellite Nusantara Satu. While the satellite was the main payload of the mission, as is common on the Falcon 9, the rocket had a few stowaways. These secondary payloads are usually experiments or spacecraft too small or too light to warrant a rocket such as CubeSats. But despite the seated positions in the economy, one of the secondary payloads of this launch has a well-timed date for fate: the Beresheet, the first mission funded by private funds to attempt to land on the moon.
But unlike the Apollo missions, which took only three days to reach our closest celestial neighbor, Beresheet takes a considerably quieter course. It will take more than a month for the spacecraft to reach the Moon and a few weeks later, it will then make a descent into the sea of serenity, not far from there. Apollo 17 landed 47 years ago. This supposes that everything goes perfectly; Add a few more weeks to this estimate if the vehicle encounters misfires on the way.
At first glance, this may seem strange. If the trip only lasted a few days with the technology of the 1960s, it seems that a modern rocket like the Falcon 9 should be able to improve its time. But in reality, the pace is dictated by the budget constraints imposed on the vehicle itself and the amplifier that transported it in space. Although it can be said that the orbital maneuvers involved in this "scenic route" to the Moon are more complicated than the direct trajectory employed by inhabited Apollo missions, they are promising for a whole new class of lunar vessels. If you are in no hurry and are trying to save money, your moon mission may be better.
Orbital mechanics of the beginner
Before we can understand the different methods used to reach the Moon, we need to know a little more about the navigation of spaceships. Specifically, how a spacecraft orbiting a body can orbit another body. As you can imagine, as he is literally a wizard, these concepts can be a bit difficult to master.
As such, we will take some liberties to make things a little easier to understand. First, let's abandon the third dimension completely and imagine that the starting and destination orbits are on the same plane. In addition, say that the orbits are well concentric, that is to say that the orbit in which we wish to pass is essentially the same as our current orbit, at a higher altitude compared to our starting position.
Obviously, the moon does not have a perfectly flat and circular orbit around the Earth, but that said, it's not a terrible analogy. Once the basics of orbital transfer are understood, there is no more progress to make to see how they could be applied to the true orbit of the moon around our planet. However, we will let the application of these concepts to more distant destinations of our solar system as an exercise for the reader.
The other important thing to understand is how the gear changes affect the orbit. As velocity increases in the direction of travel, the altitude of the orbit on the opposite the side of the burn is lifted. The altitude and position at which this speed increase occurred would then become the lowest point, or pericapsis, of a now elliptical orbit. The principle works in the same way in the opposite direction if the spacecraft were to light its engine in the opposite direction of the movement.
To put this in perspective with our 2D model, if a spacecraft gets its engine on the "right" side of the orbit, the change in altitude will occur on the "left" side. In this way, the maximum altitude of the orbit, called apoapsis, can be increased until it crosses that of the target orbit. The spacecraft then drifts along this temporary route. transfer orbit until it reaches apoapsis, where it restarts the engine to perform what is often called a "burn of circulation". This maneuver elevates the periapapsia to that of the target, thus completing the transition of the vehicle to the new orbit.
The express way
When the Apollo missions sent humans to the moon, they obviously wanted to get there as quickly as possible. Not only were the living conditions aboard the spacecraft cramped, but the longer men stayed in space, the more they would need supplies to support them. Therefore, to minimize the time spent in transit to and from the Moon, the Apollo probe was transferred directly from the low Earth orbit to the Moon's orbit.
After launch, the Apollo spacecraft was placed in a LEO parking orbit where the crew examined the vehicle's systems and prepared for the upcoming voyage. Once the probe was in the proper position, they performed the trans-lunar injection maneuver (TLI), an engine that increased their speed sufficiently to intercept the Moon's orbit. Some course corrections were made along the way, as well as braking maneuvers to slow down and enter lunar orbit, but overall, the flight path is not far removed from the simplified model that we examined earlier. .
This type of TLI maneuver is ideal because it allows you to go to the Moon as quickly as possible. It only works if the vehicle engine is powerful enough to accelerate to the required speed in the appropriate window. On the Apollo missions, the Rocketdyne J-2 engine increased the speed of the spacecraft by a little more than three kilometers per second in just six minutes.
Slow and steady
But what if you do not have the powerful J-2 engine of the Apollo spacecraft, let alone the powerful Saturn V that will transport you into space? What happens if your spaceship is the size of a bathtub and its secondary payload status prevents the booster from sending it directly to the target orbit? In this case, you need to be creative.
To reach the moon, Beresheet will eventually use several orbits to slowly raise its apoapsis in the next month. In each orbit around the Earth, as the spacecraft gets closer to the planet, it will burn the engine relatively quickly. Each etching will not only increase the apoapsis a little higher, but will also increase the orbital period (the time needed to complete an orbit). In other words, every time the engine starts, the elapsed time following the burn is pushed further. Once on the moon, the spacecraft will perform a similar operation in the opposite direction to lower itself to the lunar surface.
Because of the complex nature of these maneuvers, it's easy to understand why the trip will take so long. should Beresheet If you miss one of its scheduled burns (which it has already done), the transit time could be extended even longer. On the positive side, this allows the vehicle to reach the moon with a very small engine and gives the ground controllers all the time to adjust their course and deal with any problems.
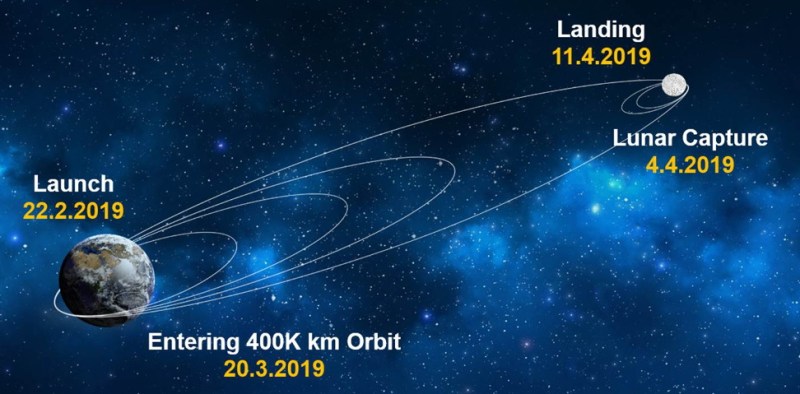
Make Luna Great Again
Despite the incredible success of inhabited Apollo missions, humanity has never taken the necessary steps to fully utilize our natural satellite. Over the next few decades, only a handful of vehicles returned to the moon and most of them never landed on the surface. But thanks to a burgeoning Chinese space program and the current administration of the United States to support its return to the moon, we could finally finish the work we had started in the 1960s.
If this is really the case, these "economic" flights to the moon could begin to become more commonplace. The launch of secondary payloads and the use of low-thrust orbital transfers could be a viable alternative to expensive direct flights. Time-sensitive payloads, such as building materials, spare parts or long-life foods, could be routinely sent to the moon with excess capacity during commercial launches; potentially creating a whole new market for the first company to develop a small general-purpose lunar cargo vehicle. Maybe one day Amazon Prime will be able to add Sea of Serenity to its list of supported locations.
[ad_2]
Source link