[ad_1]
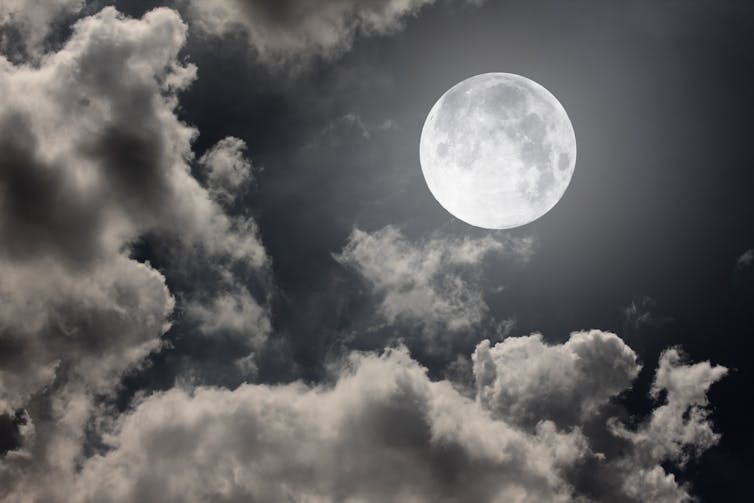
Suppakij1017 / Shutterstock
Nearly 50 years after humans first walked on the moon, the human race continues its attempts to land on Earth's satellite. This year alone, China landed on a robotic satellite on the other side of the moon, while India was preparing to land on a lunar vehicle, and Israel continues its mission of landing. on the surface, despite the crash of his recent company. NASA has announced plans to send astronauts to the South Pole of the Moon by 2024.
But as these missions seek to deepen our knowledge of the moon, we are still working to answer a fundamental question about it: how did it end up where it is?
On July 21, 1969, the crew of Apollo 11 installed the first set of mirrors reflecting lasers directed to the moon from Earth. Subsequent experiments with these networks have helped scientists calculate the distance between the Earth and the Moon in the last 50 years. We now know that the orbit of the moon has widened by 3.8 cm a year – it is moving away from the Earth.
This distance and the use of lunar rocks to date the formation of the moon 4.51 billion years ago are the basis of the hypothesis of the giant impact (the theory that the moon formed at from debris after a collision at the beginning of Earth's history). But if we assume that the lunar recession has always been 3.8 cm / year, we must go back 13 billion years to find a moment when the Earth and the Moon were close (for the moon to form). It is much too long, but the gap is not surprising and could be explained by the old continents and the tides of the world.
Tides and recession
The distance of the moon can be related to the history of continental configurations of the Earth. The loss of tidal energy (due to friction between the moving ocean and the seabed) slows the planet's rotation, forcing the moon to move away from it – the moon is moving away . Tides are largely controlled by the shape and size of the Earth's ocean basins. As Earth's tectonic plates move, the geometry of the oceans changes, as does the tide. This affects the retreat of the moon, so it appears smaller in the sky.
This means that if we know how the Earth's tectonic plates have changed position, we will be able to determine the position of the moon in relation to our planet at a given moment.
We know that the strength of the tide (and therefore the rate of recession) also depends on the distance between the Earth and the Moon. So we can assume that the tides were stronger when the moon was young and closer to the planet. As the moon quickly retreated at the beginning of its history, the tides weakened and the recession slowed down.
The detailed mathematics that describes this evolution was first developed by George Darwin, son of the great Charles Darwin, in 1880. But his formula produces the opposite problem when we introduce our modern figures. He predicted that the Earth and the Moon were close to each other only 1.5 billion years ago. Darwin's formula can be reconciled with modern estimates of the age and distance of the moon only if its typical recent recession rate is reduced to about one centimeter per year.
The implication is that the tides of today must be unusually large, resulting in a recession rate of 3.8 cm. The reason for these high tides is that the current North Atlantic Ocean offers the proper width and depth to resonate with the tide; the natural period of oscillation is thus close to that of the tide, which allows them to be very wide. . It looks a lot like a child on a swing that rises higher if it is pushed with the right timing.
But go back in time – a few million years are enough – and the shape of the North Atlantic is sufficiently different for this resonance to disappear, so that the rate of recession of the Moon will have been slower. As plate tectonics shifted continents and the slowing of Earth's rotation changed the days and tides, the planet would have crept into similar tidal states. But we do not know the details of the tides for long periods of time and, therefore, we can not tell where the moon was in the distant past.
Sediment solution
A promising approach to solving this problem is to try to detect Milankovitch cycles from physical and chemical modifications of old sediments. These cycles result from variations in the shape and orientation of the Earth's orbit and the orientation of the Earth's axis. These have produced climatic cycles, such as the ice ages of the last million years.
Most of Milankovitch's cycles do not change period in the course of Earth's history, but some are influenced by the speed of rotation of the Earth and the distance to the Moon. If we can detect and quantify these particular periods, we can use them to estimate the length of day and the Earth-Moon distance at the time of sediment deposition. Until now, this has only been attempted for a single point in the distant past. Sediments from China suggest that 1.4 billion years ago, the Earth-Moon distance was 341,000 km (its current distance is 384,000 km).
We now wish to repeat these calculations for sediments located in hundreds of locations at different times. This will provide a robust and nearly continuous record of the lunar recession over the last billion years and will allow us to better understand the evolution of the tides in the past. Together, these interdependent studies will provide a coherent picture of the evolution of the Earth-Moon system over time.
Mattias Green, Physical Oceanography Reader, Bangor University and David Waltham, Professor of Geophysics, Royal Holloway
This article is republished from The Conversation under a Creative Commons license. Read the original article.
[ad_2]
Source link