[ad_1]
The standard model of particle physics is one of the most impressive scientific achievements. It is a rigorous and precise effort to understand and describe three of the four fundamental forces of the Universe: the electromagnetic force, the strong nuclear force and the weak nuclear force. Gravity is absent because, until now, its integration into the standard model has proved extremely difficult.
However, the standard model has shortcomings, one of which concerns the neutrino mass.
The existence of the neutrino was first proposed in 1930, then detected in 1956. Since then, physicists have learned that there are three types of neutrinos: they are abundant and elusive. Only special installations can detect them because they rarely interact with other materials. They have several sources and some of them roam the space from the Big Bang, but most neutrinos close to Earth come from the Sun.
The standard model predicts that neutrinos do not have mass, like photons. But physicists have found that the three types of neutrinos can be transformed one by one. According to physicists, they should only be able to do that if they have a mass.

But how much mass? This is an issue of concern to particle physicists. And answering this question is part of what motivates KATRIN scientists (Karlsruhe Tritium Neutrino experiment.)
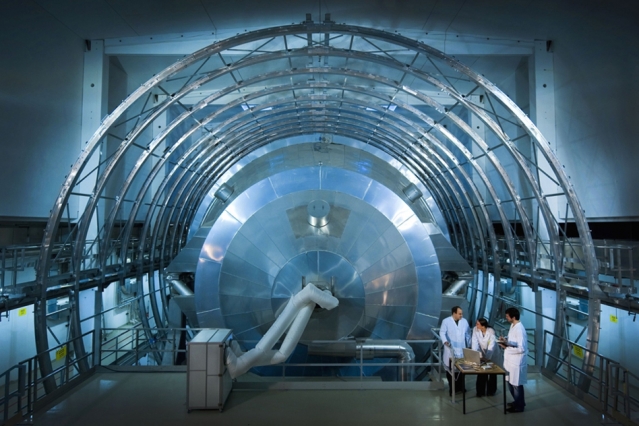
"These results from the KATRIN collaboration reduce the previous mass range of the neutrino by a factor of two …"
HAMISH ROBERTSON, SCIENTIST KATRIN AND PROFESSOR EMERITUS OF PHYSICS AT THE UNIVERSITY OF WASHINGTON.
A team of researchers has found an answer to this question: the mass of the neutrino can not exceed 1.1 electron-volts (eV). This is a reduction of the upper limit of the mass of a neutrino by nearly 1 eV; from 2 eV to 1.1 eV. Based on previous experiments setting the mass limit below 0.02 eV, these researchers defined a new range for neutrino mass. It shows that a neutrino has less than 1/500 000 of the mass of an electron. This is an important step in the advancement of the standard model.
"Knowledge of the neutrino mass will allow scientists to answer fundamental questions in cosmology, astrophysics and particle physics …"
Hamish Robertson, KATRIN scientist and professor emeritus of physics at the University of Washington.
The researchers behind this work come from 20 different research institutions around the world. They work with KATRIN at the Karlsruhe Institute of Technology in Germany. The installation KATRIN is equipped with a high resolution spectrometer of 10 meters that allows it to measure the energies of electrons with high accuracy.
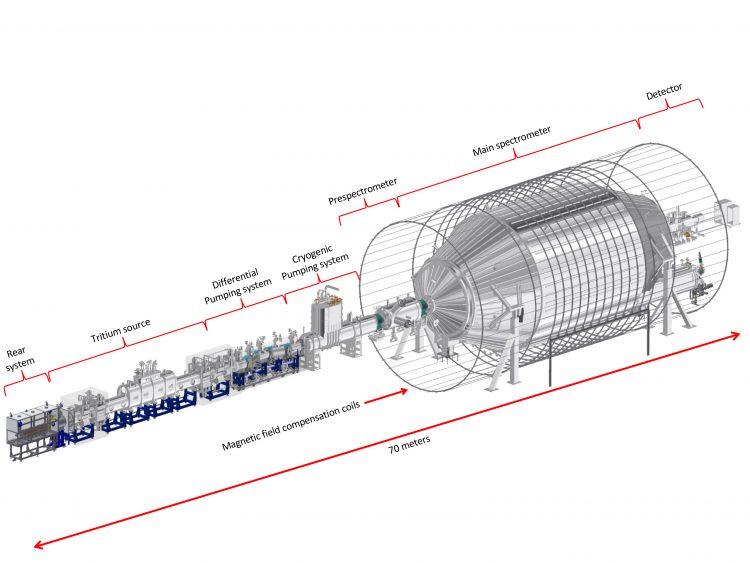
The KATRIN team presented its findings at the 2019 Topics in Astroparticle and Underground Physics conference in Toyama, Japan, on September 13th.
"Knowing the neutrino mass will allow scientists to answer fundamental questions in cosmology, astrophysics and particle physics, such as the evolution of the universe or physics going beyond the standard model," said Hamish Robertson , KATRIN scientist and professor emeritus of physics. at the University of Washington. "These discoveries from the KATRIN collaboration reduce the previous neutrino mass range by twice, set stricter criteria for the actual mass of the neutrino, and provide a way forward to measure its value in a definitive way."
Neutrinos are notoriously difficult to detect, even if they are abundant. Only photons are more abundant. As their name suggests, they are electrically neutral. This makes their detection extremely difficult. There are neutrino observatories sunk deep in the Antarctic ice, as well as in abandoned mines. They often use heavy water to induce neutrinos to interact. When a neutrino interacts, it produces a Cherenkov radiation that can be measured.
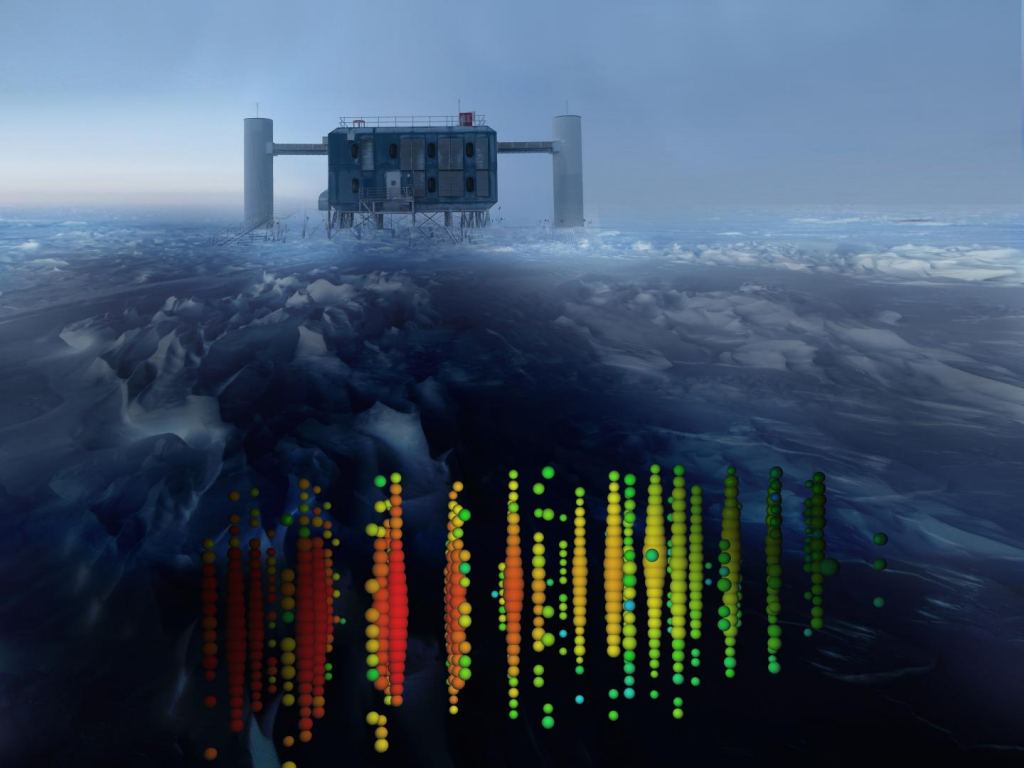
"If you filled the solar lead system fifty times beyond Pluto's orbit, about half of the neutrinos emitted by the sun would still leave the solar system without interacting with that lead," Robertson said.
The history of neutrino has evolved over time with experiments such as KATRIN. Originally, the standard model predicted that neutrinos would not have mass. But in 2001, two different detectors showed that their mass is non-zero. The 2015 Nobel Prize in Physics was awarded to two scientists who showed that neutrinos can oscillate between types, thus showing their mass.
The installation KATRIN measures the mass of neutrinos indirectly. It works by monitoring the disintegration of tritium, a highly radioactive form of hydrogen. When the isotope of tritium decays, it emits pairs of particles: an electron and an anti-neutrino. Together they share 18,560 eV of energy.
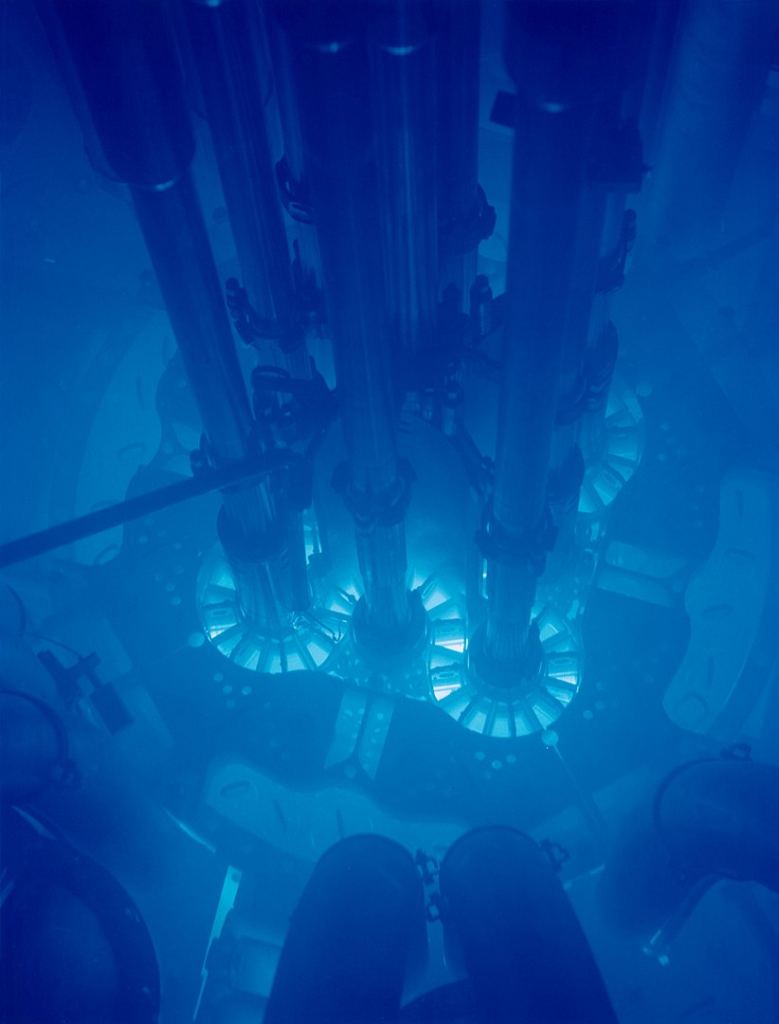
In most cases, the particle pair also shares the 18,560 eV. But in rare cases, the electron absorbs the bulk of the energy, leaving the neutrino with very little. These are the rare cases that scientists focus on.
Because of E = mC2, the minute amount of energy left to the neutrino in these rare cases must also be equal to its mass. Because KATRIN has the power to measure the electron accurately, it is also able to determine the mass of the neutrino.
"Solving the neutrino mass would take us into a new world of creating a new standard model," said Peter Doe, professor-researcher in physics at the University of Washington and working on Katrin.
This new standard model mentioned by Doe could potentially account for dark matter, which constitutes the essence of matter in the universe. Efforts such as KATRIN may one day be able to detect another fourth neutrino called sterile neutrino. Until now, this fourth type is only conjecture, but it's a candidate for dark matter.

"Neutrinos are strange small particles," said Doe. "They are so ubiquitous and we can learn a lot once we have determined this value."
It is important to demonstrate that neutrinos have mass and limit their range. But particle physicists still do not know how they are gaining mass. It's probably different from the way other particles get theirs.
Results like those of KATRIN help fill a void in the standard model and in our global understanding of the universe. The universe is full of ancient neutrinos from the Big Bang and every progress in neutrino mass helps us understand how the universe has formed and evolved.
More:
[ad_2]
Source link