[ad_1]
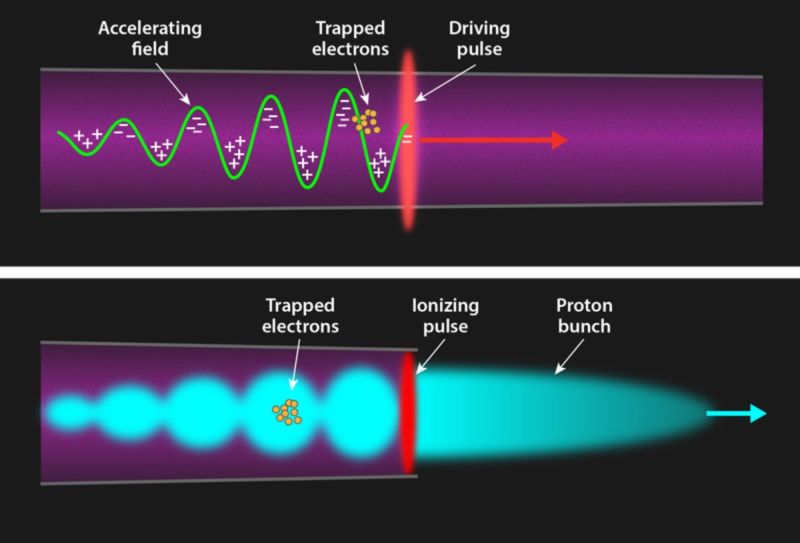
Particle accelerators can accelerate subatomic particles almost at the speed of light. The trade-off is that it requires tunnels several miles long, such machines are usually huge and very expensive to build. Physicists want to build a viable table version capable of accelerating particles in just a few centimeters. Researchers at the Lawrence Berkeley National Laboratory have now reached the highest energy ever recorded with these "plasma standby field accelerators" and describe their work in a new article from Letters of physical examination.
Conventional particle accelerators use modulated electric fields inside metal cavities to accelerate electrons. The large CERN hadron collider in Switzerland is the largest ever built, with a ring of superconducting magnets of a length of 16 km used to amplify the electrons at speeds close to light. On the other hand, the acceleration of the plasma field in the plasma involves triggering very short bursts of laser light in a cloud of ionized gas (plasma).
The resulting "wave" waving through the plasma leaves a "wake" of charged particles behind it, just like a motorboat will create a wake of water when it zooms into a lake. Then a second laser sends more electrons into the plasma. If done at the right time, these electrons can "surf" along the wake field. The electrons draw on the energy of the wake field to gain more speed, just as a surfer can gain speed by browsing the face of a wave.
Many groups are working on accelerating the wake of the plasma, using various techniques. Earlier this month, CERN's proton-driven proton-driven wakefield experiment (AWAKE) experiment uses a group of high-energy protons as the engine to create the wake, rather than the A laser pulse like the LBNL experiment – was able to accelerate the electrons to 2 GeV. AWAKE is a little behind the Berkeley team in this regard, but the fact that two different methods of plasma wake acceleration are progressing so well bodes well for the field.
Table accelerators are very promising for practical applications in medical therapies, X-ray imaging and perhaps even security scanner technologies. In fact, they are so promising that in 2015, the Gordon and Betty Moore Foundation awarded a $ 13.5 million grant to Stanford University to develop a box-size "chip-on-chip" by 2020. In bathrooms and laptops, tablets and smart phones, the idea is to use micro-fabrication techniques to build a portable particle accelerator (although the associated radiation would make it impossible to hold the device by hand).
This last document builds on the previous work of team leader Wim Leemans at LBNL; He is now director of accelerators at DESY in Germany. In 2014, he and his team reached a record acceleration of 4.25 gig-electron-volts (GeV). They used an electric shock to create a plasma from gas contained in a short, thin tube. Then, they injected a pulse of laser light to create a channel in the ionized gas to contain the pulse, in the same way that fiber optic cables channel the light. They have also created waves that trap free electrons and accelerate them at high energies.
Such an achievement requires a lot of precision and control over the laser beam, as it involves pulsing it through a 500-micron hole at a good distance of 45 feet. Fortunately, LBNL has one of the most powerful and accurate lasers in the world in Berkeley Lab Laser Accelerator (BELLA). However, although the 2014 experiment was a success, the laser light was so powerful that it continually destroyed the structure of the sapphire tube and thus lost all its precision.
Leemans et al. knew that they would have to create less dense plasma channels in the middle to reach even higher energies. They modified their installation a bit more, borrowing a technique from the 1990s: emitting an eight nanosecond laser pulse into the tube just after the discharge (420 nanoseconds after, to be precise). The pulse would heat the plasma and form a deeper channel capable of completely confining the laser. This allowed to use a tube longer than 20 cm, compared to the 9 cm tube used in the 2014 experiment.
G Bagdasarov / Keldysh Institute of Applied Mathematics; A. Gonsalves, J.L. Vay / LBNL
"The development of stable plasma acceleration with energies close to 10 GeV is an important step on the road from the laboratory to the first applications," Leemans said. "We have developed a new concept in the toolbox and, coupled with other concepts of acceleration, beam stability and beam control existing at DESY, this will create sources of energy. compact electrons. "
But we are not there yet. Written at PhysicsFlorian Grüner, from the University of Hamburg, notes that the decisive achievement of 100 MeV energies in 2004 has essentially launched this new field of acceleration of the plasma standby field. He compared this step to the publication of Jules Verne's 1865 novel, From the Earth to the moon, and the first human in space that followed in 1961 launched the era of space exploration. But he thinks we have not yet reached the equivalent of the 1969 lunar landing
"It's still unclear when" the moon landing "will occur in the form of a wakefield accelerator user facility," writes Grüner. "The key to achieving this goal will be to develop an unprecedented level of control over all relevant parameters.This means introducing brand new" buttons "for things like final energy and propagation of the Accelerated particle energy. "
DO I: Letters of physical examination, 2019. 10.1103 / PhysRevLett.122.054802 (About DOIs).
[ad_2]
Source link