[ad_1]
The first cells on the ancient Earth may have emerged because blocks of protein stabilized the membranes.
Life on Earth was born about 4 billion years ago when the first cells formed within a primordial soup of complex, carbon-rich chemical compounds.
These cells were facing a chemical enigma. They needed particular ions of soup to be able to perform the basic functions. But these charged ions would have disrupted the simple membranes that encapsulated the cells.
A team of researchers from the University of Washington solved this puzzle using only molecules that would have been present on the primitive Earth. Using compartments filled with fluid and the size of a cell, surrounded by membranes composed of fatty acid molecules, the team discovered that amino acids, building blocks of proteins, can stabilize membranes against magnesium ions. Their results paved the way for the first cells that code their genetic information into RNA, a DNA-related molecule that requires magnesium for its production, while maintaining membrane stability.
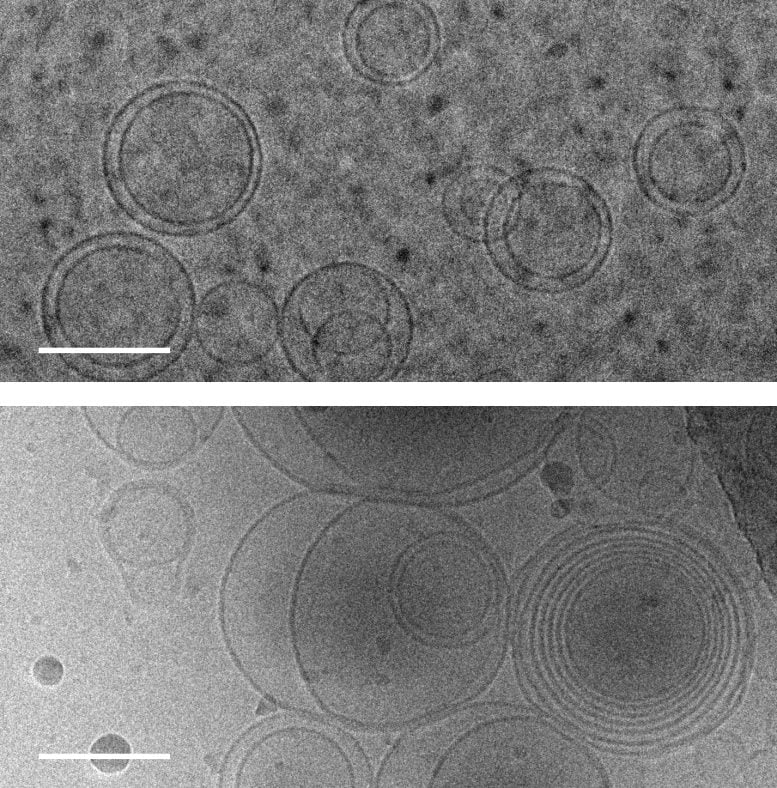
Images of membranes (circles) taken by transmission electron cryomicroscopy. Top: membranes in a solution containing no amino acids. Bottom: membranes in a solution containing serine, an amino acid, which triggers the formation of several layers of concentric membranes. Scale bars: 100 nanometers. Credit: Alex Mileant / Caitlin Cornell
The findings, published Aug. 12 in the Proceedings of the National Academy of Sciences, go beyond the explanation of how amino acids could have stabilized membranes in adverse environments. They also demonstrate how the different building blocks of cellular structures – membranes, proteins and RNA – could have been co-located in aqueous environments on the ancient Earth.
"The cells are made up of very different types of structures with totally different types of building blocks, and you never really know why they would functionally unite," said Roy Black, co-author, professor, author chemistry affiliated with UW. and bioengineering. "The hypothesis was just that – in a way – they came together."
After a career at Amgen, Black came to UW to capture the crucial details that are missing behind this "something". He teamed up with Sarah Keller, professor of chemistry at UW and expert in membranes. Black had been inspired by the observation that fatty acid molecules can self-assemble to form membranes, and hypothesized that these membranes could provide a favorable surface for assembly of building blocks of RNA and proteins.
"You can imagine different types of molecules moving in the primordial soup in the form of fuzzy tennis balls and hard squash balls bouncing into a big box being shaken," said Keller, co-author. corresponding on the paper. "If you cover a surface inside the box with velcro, only the tennis balls will stick to this surface and they will be close together. Roy was convinced that local concentrations of molecules could be improved by a similar mechanism. "
The team had previously shown that the building blocks of RNA preferentially bind to fatty acid membranes and, surprisingly, also stabilized fragile membranes against the harmful effects of salt, a common compound on Earth today.
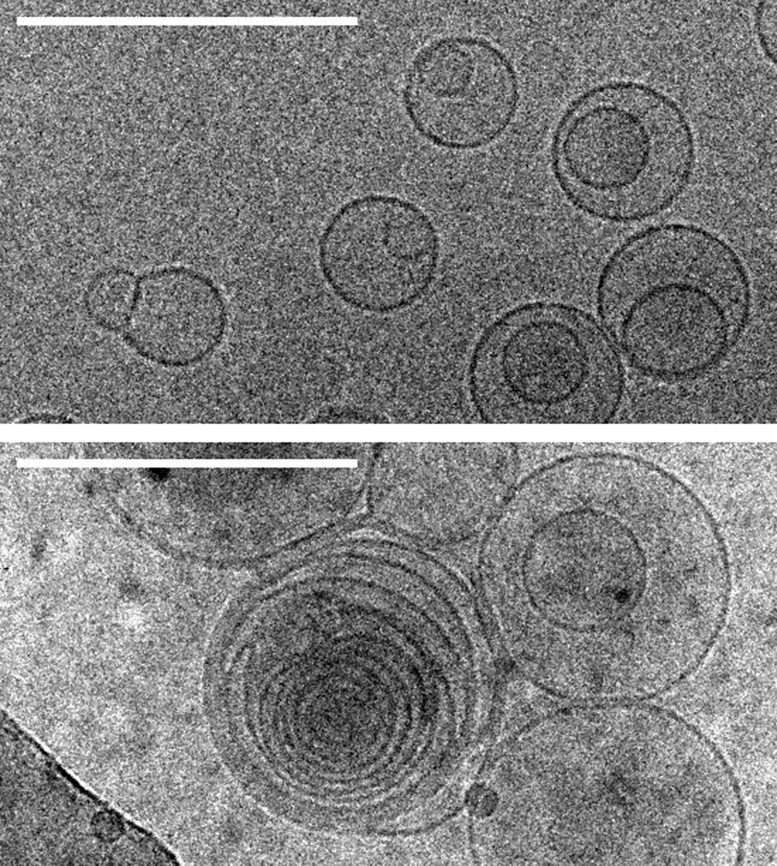
Images of membranes (circles) taken by transmission electron cryomicroscopy. Above: membranes in a solution of magnesium chloride, a salt that disrupts the membranes, without amino acids. Bottom: membranes in a solution of magnesium chloride and serine, an amino acid, which causes the membranes to form several layers of concentric membranes. Scale bars: 100 nanometers. Credit: Alex Mileant / Caitlin Cornell
The team hypothesized that amino acids could also stabilize the membranes. They used various experimental techniques (optical microscopy, electron microscopy and spectroscopy) to test the interaction of 10 different amino acids with membranes. Their experiments revealed that some amino acids bind to membranes and stabilize them. Some amino acids have even triggered important structural changes in membranes, such as the formation of concentric spheres of membranes, much like the layers of an onion.
"Amino acids did not only protect vesicles from magnesium ion disturbances, they also created multilayer vesicles, similar to nested membranes," said lead author Caitlin Cornell, a PhD student in the Department of Chemistry. University of the University.
The researchers also found that amino acids stabilized membranes by altering the concentration. Some scientists have hypothesized that the first cells may have formed in shallow basins that have undergone high and low amino acid cycles during water evaporation and during installation. new water.
New discoveries that amino acids protect membranes – as well as earlier results showing that RNA constituents may play a similar role – indicate that membranes may have been a suitable site for colocalization of these molecules. precursors, thus providing a potential mechanism for explaining what brought the ingredients together for life.
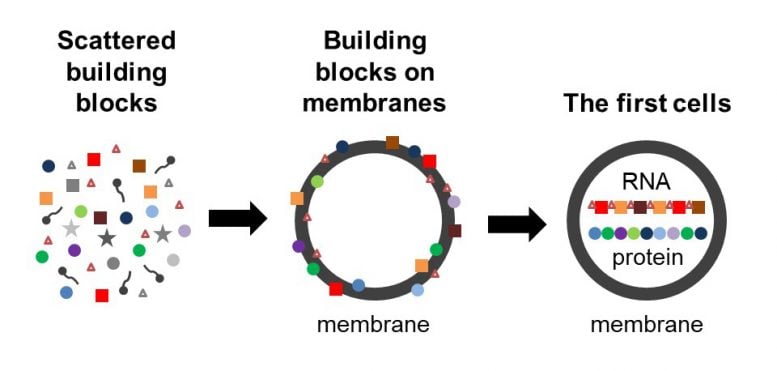
A model of how the building blocks of the first cells can be co-located on membranes. Left: the building blocks of membranes, RNA and proteins in the primordial soup. In the middle: the membranes form (gray circle) and bind a subset of the building blocks, which in turn stabilize the membranes. Right: Functional RNA and membrane-encapsulated proteins. Credit: Roy Black / Sarah Keller
Keller, Black and their team will look at how co-located building blocks have produced something even more remarkable: they have bonded to form functional machines.
"It's the next step," Black said.
Their ongoing efforts are also helping to forge links between the disciplines of the University.
"The University of Washington is a great place for discovery because of the enthusiasm of the scientific community to work collaboratively to share equipment and ideas across departments and domains," said Keller. "Our collaborations with the Drobny Lab and the Lee Lab were essential. No laboratory could do everything.
The co-authors are Gary Drobny, professor of chemistry at the University of Washington; Kelly Lee, Associate Professor of Medicinal Chemistry at the University of Washington; Postdoctoral researchers UW, Mengjun Xue and Helen Litz, from the Department of Chemistry, and James Williams, from the Department of Medicinal Chemistry; Zachary Cohen from the Department of Chemistry and Alexander Mileant from the Graduate Program in Biological Structure, Physics and Design; and Andrew Ramsay and Moshe Gordon, former undergraduate students at the University of Waterloo. The research was funded by NASA, the National Institutes of Health and the National Science Foundation.
[ad_2]
Source link