[ad_1]
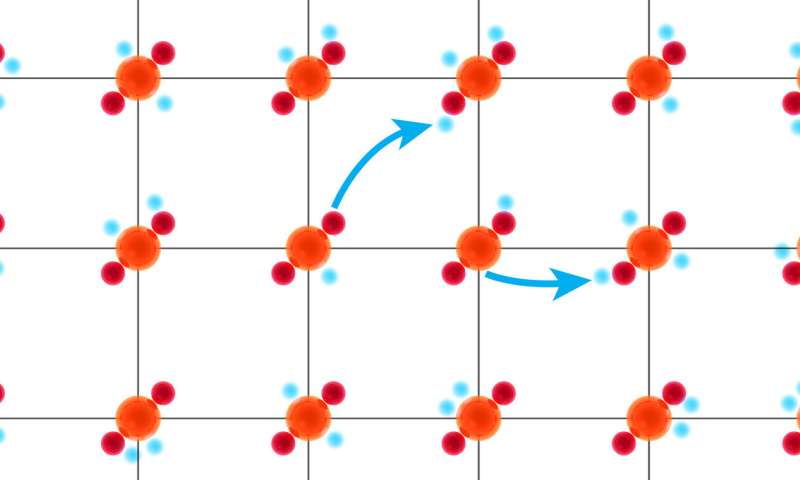
Computer simulations at SLAC and Stanford suggest a way to turn on and off superconductivity in copper-based materials called cuprates: modify the chemistry of materials so that electrons jump from atom to atom according to a particular motive – as if they were diagonally jumping to the street rather than the next to the street atom. This grid of simulated atoms illustrates the idea. The copper atoms are in orange, the oxygen atoms in red and the electrons in blue. Credit: Greg Stewart / SLAC National Accelerator Laboratory
Researchers from Stanford University and the SLAC National Accelerator Laboratory of the Department of Energy said they found the first long-sought evidence that a scientific model of the behavior of old materials from several decades could be used to simulate and understand superconductivity at high temperatures – an important step towards producing and controlling this confusing phenomenon at will.
The simulations they executed, published in Science suggest today that researchers can activate and deactivate superconductivity in copper-based materials, called cuprates, by changing their chemical composition so that electrons jump atom to atom in a particular pattern – as if they were jumping atom diagonally across the street rather than towards the next.
"The big thing you want to know is how to run the superconductors at higher temperatures and how to make them more robust," said Thomas Devereaux, co-author of the study, director of SIMES (Stanford Institute for Materials Science and Energy Sciences) of SLAC. "It's about finding the buttons that you can use to tip the scales in your favor."
The major obstacle to this task, he said, is the absence of a model – a mathematical representation of the functioning of a system – describing this type of superconductivity, whose discovery in 1986 gave hope that electricity would one day transmitted without loss of time. electric lines and maglev trains perfectly effective.
Scientists thought that the Hubbard model, used for decades to represent the behavior of electrons in many materials, could be applied to high-temperature cuprate superconductors. Until now, they had no proof, said Hong-Chen Jiang, a scientist at SIMES and co-author of the book. report.
"This has been a major unresolved problem in the field – does the Hubbard model describe high temperature superconductivity in cuprates, or is it missing a key ingredient?" he said. "Since there are several competing states in these materials, we need to rely on unbiased simulations to answer these questions, but computational problems are very difficult and progress has been slow."
The many facets of quantum materials
Why so difficult?
While many materials behave in a very predictable way – copper is always a metal and when you destroy a magnet, the bits are always magnetic – the high temperature superconductors are quantum materials, in which the electrons cooperate to produce properties unexpected. In this case, they associate to conduct electricity without resistance or loss at much higher temperatures than the established theories of superconductivity can explain.
Unlike everyday materials, quantum materials can accommodate multiple phases, or states of matter, at a time, said Devereaux. For example, a quantum material may be metallic in a set of conditions, but insulating under slightly different conditions. Scientists can tilt the balance between phases by tinkering, for example, with the chemistry of the material or the way its electrons move. The goal is to do it deliberately to create new materials with useful properties.
One of the most powerful algorithms for modeling such situations is known as Density Matrix Renormalization Group, or DMRG. But because these coexisting phases are so complex, using DMRG to simulate them requires a lot of computing time and memory, and usually takes a long time, Jiang said.
To reduce computing time and achieve a deeper level of analysis than it would have been practical before, Jiang looked for ways to optimize the simulation details. "We need to carefully rationalize every step," he said, "making it as efficient as possible and even finding a way to do two different things at the same time." These efficiency gains allowed the team to simulate the Hubbard model by DMRG much faster than before, with about a year of computing time in the Stanford Sherlock compute cluster and the Other facilities on the SLAC Campus.
Neighboring electron jumps
This study focuses on the delicate interaction between two known phases of cuprates: high temperature superconductivity and charge bands, which look like an electron density wave pattern. upper and lower in the material. The relationship between these states is unclear, with some studies suggesting that chargebands favor superconductivity and others suggesting that they compete with it.
For their analysis, Jiang and Devereaux created a virtual version of a cuprate on a square lattice, similar to a square-hole wire fence. The atoms of copper and oxygen are confined to the planes in the real material, but in the virtual version they become unique and virtual atoms located at each of the intersections where the threads meet. Each of these virtual atoms can receive at most two electrons that are free to jump or jump, either to their immediate neighbors on the square array, or diagonally across each square.
When the researchers used DMRG to simulate the Hubbard model as it was applied to this system, they discovered that modifications to the electron jump patterns had a noticeable effect on the relationship between charge bands and superconductivity .
When the electrons jumped only to their immediate neighbors on the square array, the pattern of charge bands became stronger and the superconducting state never appeared. When the electrons were allowed to jump diagonally, the charge bands finally weakened, but did not leave and the superconducting state finally emerged.
"Until now, we could not go far enough in our modeling to see if chargebands and superconductivity could coexist when this material is in its lowest energy state.We know it now, at least for systems of this size, "said Devereaux.
The question of whether the Hubbard model describes all the incredibly complex behavior of real cuprates is still open, he added. Even a small increase in the complexity of the system would require a considerable jump in the power of the algorithm used to model it. "The time required for simulation increases exponentially with the width of the system you want to study," Devereaux said. "It's exponentially more complicated and more demanding."
But with these results, he said: "We now have a fully interacting model that describes high temperature superconductivity, at least for size systems that we can study, which is a big step forward."
Two advances in understanding the role of "loadbands" in superconducting stresses
"Superconductivity in the doped Hubbard model and its interaction with the next nearest jump" Science (2019). science.sciencemag.org/cgi/doi… 1126 / science.aal5304
Quote:
Scientists finally find superconductivity in place for decades (September 26, 2019)
recovered on September 26, 2019
at https://phys.org/news/2019-09-scientists-superconductivity-decades.html
This document is subject to copyright. Apart from any fair use for study or private research purposes, no
part may be reproduced without written permission. Content is provided for information only.
[ad_2]
Source link