[ad_1]
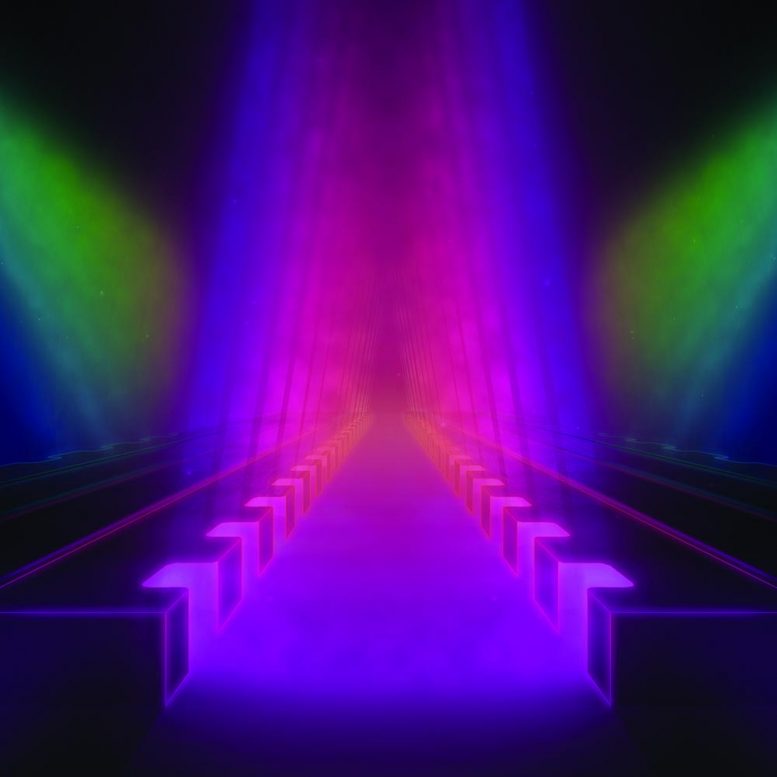
An artistic rendering of a high Q metasurface beam splitter. These “high quality factor” or “high Q” resonators could lead to new ways of manipulating and using light. Credit: Riley A. Suhar
Researchers have designed ultra-thin silicon nanoantennas that trap and redirect light, for applications in quantum computing, LIDAR and even virus detection.
The light is notoriously fast. Its speed is crucial for a rapid exchange of information, but as light passes through materials its chances of interacting and exciting atoms and molecules can become very low. If scientists could curb light particles, or photons, it would open the door to a host of new technological applications.
Now, in an article published on August 17, 2020, in Nanotechnology of nature, Stanford scientists demonstrate a new approach to slowing down light significantly, just as an echo chamber holds sound and directs it at will. Researchers in the lab of Jennifer Dionne, associate professor of materials science and engineering at Stanford, structured ultra-thin silicon chips into nanoscale bars to resonantly trap light, then release or redirect it later. These “high quality factor” or “high Q” resonators could lead to new ways of manipulating and using light, including new applications for quantum computing, virtual reality and augmented reality; WiFi light based; and even virus detection like SARS-CoV-2.
“We’re basically trying to trap the light in a little box that still allows the light to come and go in many different directions,” said postdoctoral fellow Mark Lawrence, who is also the lead author of the article. “It’s easy to trap light in a multi-sided box, but not so easy if the sides are transparent – as is the case with many silicon-based applications.”
Make and manufacture
Before you can manipulate light, resonators have to be fabricated, which poses a number of challenges.
A central component of the device is an extremely thin layer of silicon, which traps light very efficiently and has low absorption in the near infrared, the spectrum of light that scientists want to control. The silicon sits on a wafer of transparent material (sapphire, in this case) in which the researchers direct an electron microscope “pen” to etch their nanoantenna pattern. The pattern should be drawn as evenly as possible, as these antennas serve as walls in the echo chamber analogy, and imperfections inhibit the ability to trap light.
“High quality resonances require the creation of extremely smooth sidewalls that do not allow light to escape,” said Dionne, who is also deputy vice director of research platforms / shared facilities. “This can be done fairly regularly with larger micron scale structures, but it’s very difficult with nanostructures that scatter more light.”
Pattern design plays a key role in the creation of high-Q nanostructures. “On a computer, I can draw ultra-smooth lines and blocks of any given geometry, but the fabrication is limited,” Lawrence said. “In the end, we had to find a design that offered good light trapping performance, but fell within the realm of existing manufacturing methods.”
High quality (factor) applications
The tinkering with the design resulted in what Dionne and Lawrence describe as an important platform technology with many practical applications.
The devices have demonstrated quality factors of up to 2,500, which is two orders of magnitude (or 100 times) higher than any similar device obtained previously. Quality factors are a measure describing the resonance behavior, which in this case is proportional to the lifetime of the light. “By achieving quality factors in the thousands, we are already in an ideal position for some very interesting technological applications,” Dionne said.
For example, biological detection. A single biomolecule is so small that it is essentially invisible. But passing light on a molecule hundreds or thousands of times can dramatically increase the chances of creating a detectable scattering effect.
Dionne’s laboratory is working on the application of this technique to detection COVID-19[feminine antigènes – molécules qui déclenchent une réponse immunitaire – et anticorps – protéines produites par le système immunitaire en réponse. «Notre technologie donnerait une lecture optique comme les médecins et les cliniciens ont l’habitude de voir», a déclaré Dionne. “Mais nous avons la possibilité de détecter un seul virus ou de très faibles concentrations d’une multitude d’anticorps en raison des fortes interactions lumière-molécule.” La conception des nanorésonateurs à Q élevé permet également à chaque antenne de fonctionner indépendamment pour détecter différents types d’anticorps simultanément.
Bien que la pandémie ait stimulé son intérêt pour la détection virale, Dionne est également enthousiasmée par d’autres applications, telles que LIDAR – ou Light Detection and Ranging, qui est une technologie de mesure de distance au laser souvent utilisée dans les véhicules autonomes – que cette nouvelle technologie pourrait apporter à. «Il y a quelques années, je n’aurais pas pu imaginer les immenses espaces d’application que ce travail toucherait», a déclaré Dionne. «Pour moi, ce projet a renforcé l’importance de la recherche fondamentale – vous ne pouvez pas toujours prédire où la science fondamentale va aller ou ce qu’elle va mener, mais il peut apporter des solutions essentielles aux défis futurs.»
Cette innovation pourrait également être utile en science quantique. Par exemple, diviser des photons pour créer des photons intriqués qui restent connectés à un niveau quantique même éloignés les uns des autres nécessiterait généralement de grandes expériences optiques sur table avec de gros cristaux coûteux polis avec précision. «Si nous pouvons faire cela, mais utiliser nos nanostructures pour contrôler et façonner cette lumière enchevêtrée, peut-être qu’un jour nous aurons un générateur d’enchevêtrement que vous pourrez tenir dans votre main», a déclaré Lawrence. «Avec nos résultats, nous sommes ravis d’examiner la nouvelle science réalisable maintenant, mais aussi d’essayer de repousser les limites de ce qui est possible.»
Référence: «Métasurfaces à gradient de phase de facteur de haute qualité» par Mark Lawrence, David R. Barton III, Jefferson Dixon, Jung-Hwan Song, Jorik van de Groep, Mark L. Brongersma et Jennifer A. Dionne, 17 août 2020, Nanotechnologie de la nature.
DOI: 10.1038 / s41565-020-0754-x
Les autres co-auteurs de Stanford incluent les étudiants diplômés David Russell Barton III et Jefferson Dixon, l’associé de recherche Jung-Hwan Song, l’ancien chercheur scientifique Jorik van de Groep et Mark Brongersma, professeur de science et d’ingénierie des matériaux. Ce travail a été financé par le DOE-EFRC, «Photonics at Thermodynamic Limits» ainsi que par l’AFOSR. Jen est également professeur agrégé, par courtoisie, de radiologie et membre du Wu Tsai Neurosciences Institute et de Bio-X.
[ad_2]
Source link