[ad_1]
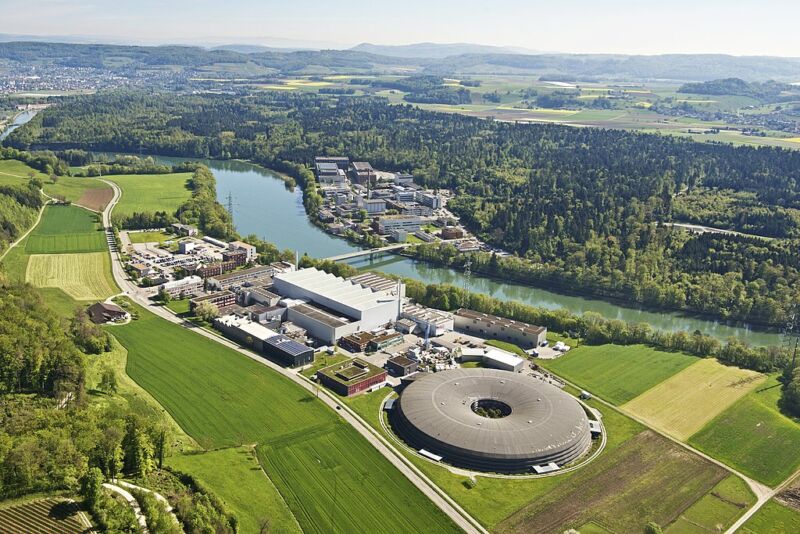
Physicists, who dedicate their lives to studying the subject, don’t seem to really like physics since they always hope it is broken. But we will have to forgive them; finding out that a little theory can’t explain experimental results is a sign that we probably need a new theory, which any physicist would excite.
In recent years, one of the things that has seemed the most broken is a seemingly simple measurement: the proton’s charge radius, which is a measure of its physical size. Measurements made with hydrogen atoms, which have a single electron orbiting a proton, gave us an answer. Measurements in which the electron was replaced by a heavier particle, called a muon, gave us a different answer – and the two results were incompatible. A lot of effort has been put into closing that gap, and it has narrowed, but it has not gone away.
This makes theorists salivate. The Standard Model doesn’t have space for these kinds of differences between electrons and muons, so could this be a sign that the Standard Model is wrong? The team behind some of the previous measurements are now back with a new one, this one following the behavior of a muon orbiting a helium nucleus. The results are consistent with other measurements of helium’s charge radius, suggesting that there is nothing funny about the muon. So the standard model can breathe a sigh of relief.
Measure muons?
The measure involved is, to put it simply, pretty insane. Muons are basically heavy versions of electrons, so it’s relatively easy to substitute them for each other in an atom. And the mass of a muon offers certain advantages for this type of measurement. The mass ensures that the muon’s orbitals become so compact that its wave function overlaps the wave function of the nucleus. As a result, the behavior of the muon when orbiting a nucleus is very sensitive to the charge radius of the nucleus.
All of this would be great if it weren’t for the fact that muons are unstable and typically decay in less than two microseconds. Orbiting a helium nucleus adds complications, as helium typically has two electrons orbiting and they can interact with each other. The three-way interactions expected of a nucleus-muon-electron are currently beyond our computational capacity, meaning we would have no idea if the actual behavior differed from theory.
The researchers therefore solved this problem by creating a positively charged ion composed of a helium nucleus and a single muon orbiting around it. Making one of these – or, more accurately, making hundreds of them – is the starting point of the madness.
The researchers had access to a muon beam created by a particle collider, and they decided to direct the beam in helium gas. In this process, when muons enter, they have too much energy to orbit a helium nucleus, so they bounce back, losing energy with each collision. Once muons slow down enough, they can enter a high-energy orbit in a helium atom, blowing one of its electrons in the process. But the second electron is still there, spoiling any potential measurements.
But the muon has a lot of momentum because of its mass, and the energy transfers within an atom are faster than the energy lost to the environment. So when the muon transfers some of its energy to the electron, the smaller mass of the electron ensures that this is enough to push the electron out of the atom, and we are left with one muonic helium ion. Fortunately, all of this happens quickly enough that the muon has no chance of decaying.
Let the madness begin
At this point, the muon is usually in an orbital which has lower energy but has more energy than the ground state. The researchers set up a trigger sensitive to the appearance of muons in the experiment. After a delay to allow the muons to start both electrons, the trigger causes a laser to strike the sample with the right amount of energy to amplify the muon from the 2S orbital to the 2P orbital. From there, it will disintegrate into the ground state, releasing an x-ray in the process.
Many muons will not be in a 2S orbital and the laser will have no effect on them. The researchers were willing to sacrifice much of the muonic helium they were making in order to get precision measurements of those in the good condition. Their presence was signaled by the detection of an x-ray with the correct energy. To make sure they were looking at the right thing, the researchers took only data associated with a high-energy electron produced by the decay of the muon.
And remember, this all had to happen fast enough to happen within the millisecond time window before the muon decayed.
The first step was to set the laser used at the right frequency to amplify the muon in the 2P orbit, as this is the value we need to measure. This was done by adjusting a tunable laser over a range of frequencies until the helium began to produce x-rays. Once the frequency was identified, the researchers took the data for 10 days, which was enough to precision frequency measurements. During this time, the researchers observed 582 muonic helium ions.
Based on calculations using laser frequency, the researchers found that the helium core’s charge radius was 1.6782 femtometers. Measurements made by bouncing electrons off the nucleus indicate that it is 1.681. These two values are part of the experimental errors, so they are in strong agreement.
We’re sorry, it’s not broken
At the simplest level, the fact that muon measurements agree with measurements taken independently indicates that there is nothing special about muons. Therefore, the Standard Model, which says the same thing, is intact up to the small enough limits allowed by the experimental errors here. (That’s not to say it’s not broken some other way, of course.) So theorists around the world will be disappointed.
As an amusing aside, the researchers compared their value to that generated decades ago in CERN particle accelerators. It turns out that this value is similar, but only by accident, since previous work had two compensation errors. “Their quoted charge radius is not very far from our value,” the researchers note, “but this can be attributed to an awkward coincidence of a bad experiment combined with an incomplete prediction of the 2P – 2S theory, giving – false value. “So in this case two wrongs made a near-right.
Either way, this work will once again focus researchers’ attention on trying to understand why different experiments with protons keep producing results that don’t quite agree, because we can’t blame things. on the muon being weird. In the meantime, we can all appreciate how amazing it is to be able to do so much with muons in the tiny fraction of a second they exist.
Nature, 2021. DOI: 10.1038 / s41586-021-03183-1 (About DOIs).
[ad_2]
Source link