[ad_1]
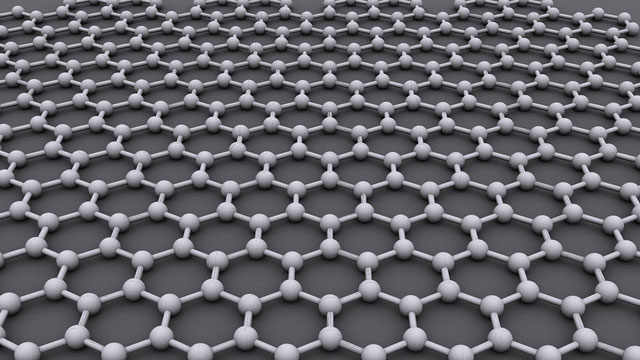
The ideal crystalline structure of graphene is a hexagonal grid.
This being Ars, I suppose many readers are familiar with Peltier coolers, which use an effect called a thermoelectric effect and use electrical energy to pump heat. The advantage of the thermoelectric effect is that it works in both directions: a difference in temperature can generate electrical energy. The problem, of course, is that electricity production in this way is about as efficient as that of a teenage household.
But if you choose the right materials, this does not have to be the case. Recent research shows that it could be possible to convert heat energy into electrical energy with a yield of between 15 and 20%. If it can Actually to be realized, it would be a truly incredible advance.
Set fire to efficiency
What makes thermoelectric conversion so inefficient? It boils down to how heat and electricity are moved through a material. The heat is transmitted through a material in two different ways. One of them passes through the vibrations of the atoms – the heat is essentially carried by sound waves called phonons. But electrons can also carry heat. In an insulator, the electrons do not go very far, the heat is only transmitted by phonons. In an electrical conductor, however, the heat transfer is more likely to be dominated by the movement of electrons.
The electrons carry energy for a certain distance, then bounce off an atom, transferring the energy to the phonons. The opposite also occurs, so the energy flows between the phonons and the electrons. This process unfolds incredibly quickly, rapidly displacing thermal energy through electrically conductive materials.
This is bad for an efficient thermoelectric conversion because you need excellent electrical conductivity and poor thermal conductivity to make sure the heat is moving the electrons. However, the relationship between the thermal conductivity of electrons and the electrical conductivity of a material is, in most cases, fixed. This leaves little room for researchers to optimize or improve thermoelectric generation.
Electrons that ignore atoms
There is an exception. The critical step is not that the electrons carry thermal energy but that they restore it to the phonons. But, in some materials, electrons do not run very often in atoms, so the transfer of electron energy into phonons is inefficient. Better still, the electrons collide a lot. This combination can result in a net charge flow in one direction (an electric current), while heat transfer is reduced by electron collisions. Graphene is a material that meets this criterion.
A team of researchers calculated how it would work for a junction between two bits of graphene. In the first calculation of the researchers, they use open circuit conditions so that no current flows. This shows that the proportion of thermal power absorbed by the electrons near the junction increases as the incoming power increases. Indeed, the electrons concentrate the heat at the junction but do not transfer energy to the phonons. This results in a very strong temperature gradient between the edges of the graphene bits and the junction.
To verify that this is really due to an inefficient transfer of energy to phonons, the researchers modify their model to add additional energy transfer to phonons. Doing this reduces the temperature gradient and there is no focusing effect (conversely, in fact, heat is spreading).
Extracting energy
Does this hold when electrons are allowed to drive and extract energy? The answer is yes, it seems. Once the circuit closed with resistance, the researchers showed that even when the residual heat transfer of phonons is taken into account, about 15% of the incoming thermal radiation is emitted as electrical energy.
To what extent is the direct conversion of heat energy into electricity realistic? Well, first of all, these are calculations. Experiments still need to be done. And there are some "traps!" in the newspaper. For example, the maximum efficiency corresponds to a heat radiation intensity of about 200 W / cm2. To put this into perspective, the radiant intensity of a heat lamp with a filament temperature of about 2700 ° C will satisfy this requirement (at a distance of 60 cm). This seems difficult to achieve in a typical use.
Then there is the material problem. Here, the researchers used graphene for their calculations. This is by far the best material available for this type of device. But to get a high efficiency, you have to cool the graphene network to -223 ° C, otherwise the phonons will start to transfer a lot of heat. Thus, unless new materials arise, high-efficiency thermoelectric power generation will be limited to two things: a very cold set of experiments in the laboratory and, perhaps, energy production for the instruments. space.
I still want to see less modeling and more experimental results, though.
Letters of physical examination, 2019, DOI: 10.1103 / PhysRevLett.122.166802 (About DOIs)
[ad_2]
Source link