[ad_1]
Welcome back to our second guided walk in the woods of quantum mechanics! Last week we saw how particles move like waves and strike like particles and how a single particle takes multiple paths. While surprising, this is a well-explored area of quantum mechanics – it can be found on the paved nature path around the visitor center.
This week I’d like to get off the paved trail and go a little deeper into the woods to talk about how particles blend and combine in motion. This is a subject generally reserved for physics majors; it is rarely discussed in popular articles. But the payoff is understanding how precision lidar works and seeing one of the great inventions coming out of the lab, the optical comb. So let’s go put on our slightly dirty (quantum) hiking boots – it will be worth it.
Two particles
Let’s start with a question: if the particles move like waves, what happens when I overlap the paths of two particles? Or put another way, do particle waves interact only with themselves or do they mix?
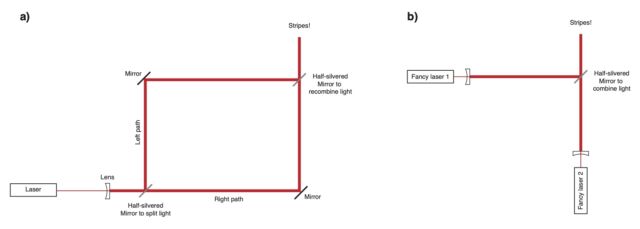
Miguel Morales placeholder image
We can test this in the lab by changing the setup we used last week. Instead of splitting the light from a laser into two paths, we can use two separate lasers to create the light entering the final semi-silver mirror.
We have to be careful with the lasers we use, and the quality of your laser pointer is no longer up to the task. If you carefully measure the light from a normal laser, the color of the light and the phase of the wave (when the wave peaks occur) will err. This color wandering isn’t noticeable to our eyes – the laser always looks red – but it turns out that the exact shade of red varies. This is a problem that money and modern technology can solve – if we shell out enough money, we can buy precision mode lock lasers. Thanks to these, we can have two lasers both emitting photons of the same color with wave peaks aligned in time.
When we combine the light from two high quality lasers, we see the exact same pattern of stripes that we have seen before. Particle waves produced by two different lasers interact!
So what if we go back to the single photon limit? We can reduce the intensity of the two lasers so low that we see the photons appear one by one on the screen, like little paintballs. If the rate is low enough, only one photon will exist between the lasers and the screen at a time. When we do this experiment, we will see the photons arrive on the screen one by one; but when we look at the accumulated pointillism paint, we will see the same stripes we saw last week. Once again, we see interference from single particles.
It turns out that all of the experiments we’ve done before give the exact same answer. Nature doesn’t care if a particle interacts with itself or if two particles interact with each other – a wave is a wave, and particle waves act like any other wave.
But now that we have two precision lasers, we have a number of new experiments that we can try.
Two colors
First, let’s try interfering photons of different colors. Let’s take the color of one of the lasers and make it slightly bluer (shorter wavelength). When we look at the screen we see stripes again, but now the stripes are slowly walking to the side. The appearance of the stripes and their movement are interesting.
First, the fact that we are seeing stripes indicates that particles of different energy are still interacting.
The second observation is that the striped pattern is now time dependent; the stripes walk on the side. As we enlarge the color difference between lasers, the speed of the scratches increases. Audience musicians will already recognize the beat pattern we see, but before we get to the explanation, let’s improve our experimental setup.
If we just use narrow laser beams, we can use a prism to combine the light fluxes. A prism is typically used to split a single beam of light and send each color in a different direction, but we can use it upside down and with careful alignment use the prism to combine the light from two lasers into one beam. .
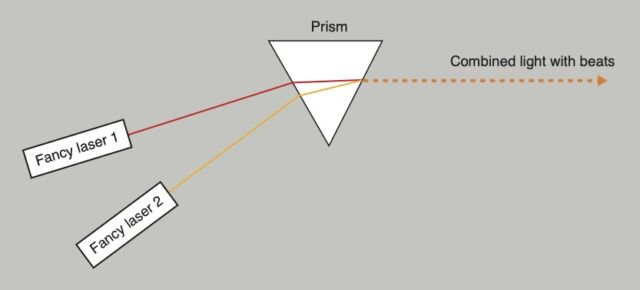
Miguel Morales placeholder image
If we look at the intensity of the combined laser beam, we will see the intensity of the “beat” of the light. While the light from each laser was constant, when their slightly different colored beams are combined, the resulting beam oscillates from light to dark. Musicians will recognize this by tuning their instruments. When the sound of a tuning fork is combined with the sound of a slightly detuned string, the “beats” can be heard as the sound oscillates between loud and soft. The beat speed is the difference in frequencies and the string is tuned by setting the beat speed to zero (zero frequency difference). Here we see the same with light – the beat frequency is the difference in color between lasers.
While this makes sense when you think of instrument strings, it’s quite surprising when you think of photons. We started off with two steady streams of light, but now the light is clustered between times when it’s bright and times when it’s weak. As the difference between the colors of the lasers increases (they are out of tune), the faster the pulse becomes.
Paintballs in time
So what if we turn the lasers really low again? Again, we see the photons hitting our detector one by one like little paintballs. But if we watch carefully when the photons arrive, we see that it is not random – they arrive in rhythm with the beats. It doesn’t matter how fast we spin the lasers – photons may be so rare that they only appear every 100 beats – but they will always arrive at the beat of the beats.
This pattern is even more interesting if we compare the arrival time of the photons in this experiment with the scratches we saw with our laser pointer last week. One way to understand what happens in the two-slit experiment is to imagine the wave nature of quantum mechanics indicating where photons can land side by side: paintballs can strike in bright regions, not in bright regions. dark regions. We see a similar pattern in the arrival of paintball in the two-color beam, but now the paintballs are directed forward and backward in time and can only hit in time with the beats. The beats can be thought of as stripes in time.
[ad_2]
Source link