[ad_1]
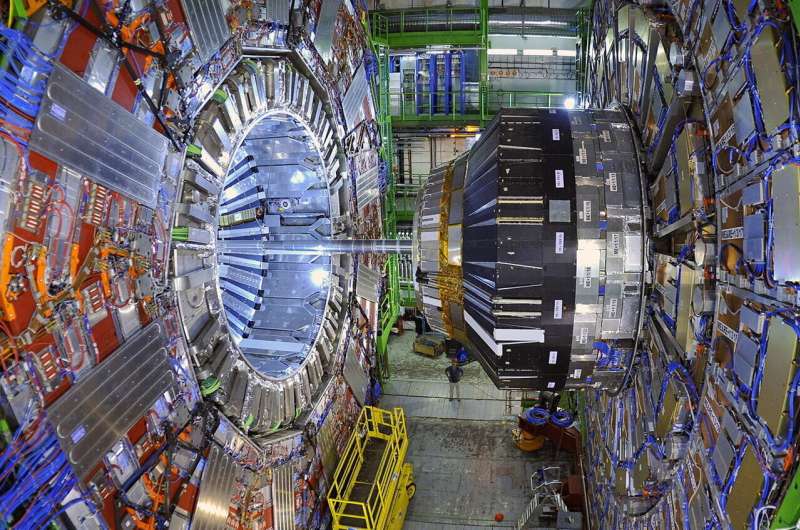
The Compact Muon Solenoid experiment at the Large Hadron Collider of the European Organization for Nuclear Research. Credit: CERN
Right after proving an 87-year-old prediction that matter can be generated directly from light, physicists at Rice University and their colleagues detailed how this process could impact future studies of primordial plasma. and physics beyond the Standard Model.
“We’re basically looking at light collisions,” said Wei Li, associate professor of physics and astronomy at Rice and co-author of the study published in Physical examination letters.
“We know from Einstein that energy can be converted into mass,” said Li, a particle physicist who is collaborating with hundreds of colleagues on experiments in high-energy particle accelerators like the Large Hadron Collider (LHC ) of the European Organization for Nuclear Research and Brookhaven National. The Laboratory’s Relativistic Heavy Ion Collider (RHIC).
Accelerators like the RHIC and the LHC regularly transform energy into matter by accelerating pieces of atoms close to the speed of light and smashing them against each other. The discovery in 2012 of the Higgs particle at the LHC is a notable example. At the time, the Higgs was the last unobserved particle in the Standard Model, a theory that describes the fundamental forces and building blocks of atoms.
As impressive as it is, physicists know that the Standard Model only explains about 4% of the matter and energy in the universe. Li said this week’s study, lead author of which was Rice’s postdoctoral researcher Shuai Yang, had implications for research in physics beyond the Standard Model.
“There are papers predicting that you can create new particles from these ionic collisions, that we have such a high photon density in these collisions that these photon-photon interactions can create new physics beyond the Standard Model.” Li said.
Yang said, “To research new physics, you have to understand the Standard Model processes very precisely. important to take this into account. “
The effect detailed by Yang and his colleagues occurs when physicists accelerate opposing beams of heavy ions in opposite directions and point the beams at each other. Ions are nuclei of massive elements like gold or lead, and ion accelerators are particularly useful for studying the strong force, which binds the fundamental building blocks called quarks in the neutrons and protons of atomic nuclei. Physicists have used heavy ion collisions to overcome these interactions and observe both quarks and gluons, the particles they exchange when they interact via the strong force.
But nuclei aren’t the only ones to collide in heavy ion accelerators. The ion beams also produce electric and magnetic fields that envelop each nucleus of the beam in its own cloud of light. These clouds move with the nuclei, and when clouds of opposing beams meet, individual particles of light called photons can meet head-on.
In a PRL A study published in July, Yang and his colleagues used data from RHIC to show that photon-photon collisions produce matter from pure energy. In the experiments, the light crushes occurred with collisions of nuclei that created a primordial soup called quark-gluon plasma, or QGP.
“At RHIC, you can make the photon-photon collision create its mass at the same time as the formation of the quark-gluon plasma,” Yang said. “So you are creating this new mass inside the quark-gluon plasma.”
Yang’s PhD thesis work on RHIC data published in PRL in 2018, it was suggested that photon collisions could affect plasma in a mild but measurable way. Li said it was both intriguing and surprising, because photon collisions are an electromagnetic phenomenon, and quark-gluon plasmas are dominated by the strong force, which is much more powerful than the electromagnetic force.
“To interact strongly with quark-gluon plasma, it is not enough to have an electric charge,” Li said. “You don’t expect it to interact very strongly with quark-gluon plasma.”
He said that a variety of theories have been proposed to explain Yang’s unexpected findings.
“One explanation offered is that the photon-photon interaction will be different not because of the quark-gluon plasma, but because the two ions come closer to each other,” Li said. “It’s related. to quantum effects and how photons interact with each other. “
If quantum effects had caused the anomalies, Yang presumed, they could create detectable interference patterns when ions narrowly miss each other but photons from their respective clouds of light collide.
“So the two ions do not collide directly,” Yang said. “They’re actually passing. It’s called an outermost collision, because the photons collide but the ions don’t collide.”
The theory suggested that the quantum interference patterns from outermost photon-photon collisions should vary in direct proportion to the distance between the passing ions. Using data from the LHC’s Compact Muon Solenoid (CMS) experiment, Yang, Li and their colleagues found that they could determine this distance, or impact parameter, by measuring something completely different.
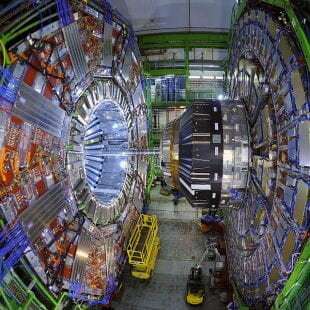
The Compact Muon Solenoid experiment at the Large Hadron Collider of the European Organization for Nuclear Research. Credit: CERN
“The two ions, as they get closer, there is a higher probability that the ion will be excited and start emitting neutrons, which descend directly along the beam line,” said Li. “We have a detector for that at CMS.”
Each outermost photon-photon collision produces a pair of particles called muons, which usually collide in opposite directions. As predicted by theory, Yang, Li and their colleagues discovered that quantum interference distorted the muon departure angle. And the shorter the distance between the quasi-mission ions, the greater the distortion.
Li said the effect comes from the movement of colliding photons. Although each moves in the direction of the beam with its host ion, photons can also move away from their hosts.
“Photons also have movement in the perpendicular direction,” he said. “And it turns out, exactly, that this perpendicular movement gets stronger as the impact parameter gets smaller and smaller.
“It looks like something is changing the muons,” Li said. “It looks like one goes at a different angle than the other, but it really isn’t. It’s an artefact. of how the photon’s motion changed, perpendicular to the direction of the beam, before the collision that created the muons. “
Yang said the study explained most of the anomalies he had previously identified. Meanwhile, the study established a new experimental tool to control the impact parameter of photonic interactions that will have large-scale impacts.
“We can comfortably say that the majority came from this QED effect,” he said. “But that does not exclude that there are still effects related to quark-gluon plasma. This work gives us a very precise basis, but we need more precise data. We still have at least 15 years to collect data. QGP data to CMS, and the data accuracy will be higher and higher. ”
Contactless creation in lead and gold core collisions
AM Sirunyan et al, Observation of Forward Neutron Multiplicity Dependence of Dimuon Acoplanarity in Ultraperipheral Pb-Pb Collisions at sNN = 5.02 TeV, Physical examination letters (2021). DOI: 10.1103 / PhysRevLett.127.122001
Provided by Rice University
Quote: Understanding Photon Collisions Could Help Research Physics Beyond the Standard Model (2021, September 20) retrieved September 20, 2021 from https://phys.org/news/2021-09-physicists-probe- smashups-future.html
This document is subject to copyright. Other than fair use for private study or research purposes, no part may be reproduced without written permission. The content is provided for information only.
[ad_2]
Source link